Natural Selection: Unveiling The Four Cornerstones Of Evolution
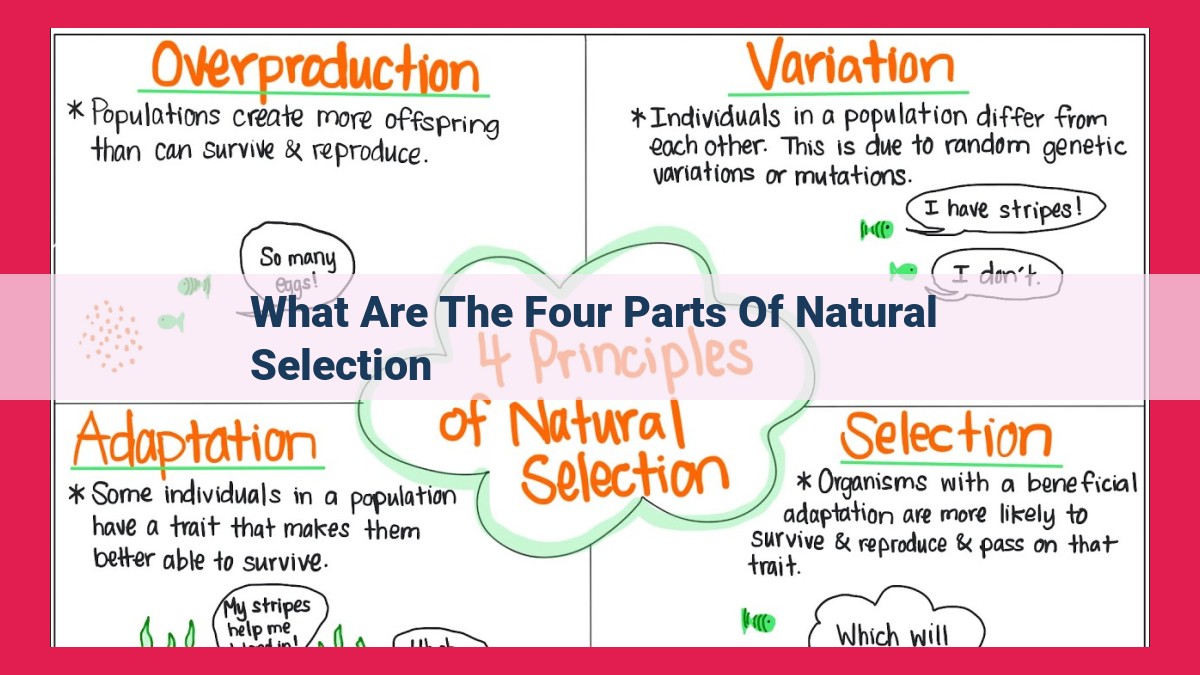
Natural selection operates through four essential parts: variation, providing the raw material for selection; inheritance, ensuring traits are passed on; selection, driving the process by favoring beneficial traits; and time, allowing for gradual evolutionary change. These interconnected components drive the evolution of life, leading to the adaptation and diversification of species.
Natural Selection: Unraveling the Mechanisms of Evolution
Evolution, a fascinating and dynamic process, is driven by the force of natural selection. To understand this enigmatic concept, let’s delve into its essential parts, beginning with variation, the cornerstone of evolutionary change.
Genetic and Phenotypic Variation: The Building Blocks of Diversity
Every organism possesses unique characteristics, a result of genetic and phenotypic variation within its population. Genetic variation arises from mutations (changes in DNA), gene recombination during meiosis, and gene flow between populations. These genetic differences translate into phenotypic variation, manifesting as observable traits that range from physical appearance to behavioral patterns.
How Variation Emerges: A Trio of Sources
Mutations, spontaneous changes in genetic material, introduce novel genetic traits. When mutations appear in reproductive cells, they can be passed on to offspring, potentially creating new heritable characteristics. Recombination, the exchange of genetic material between chromosomes during meiosis, shuffles genetic combinations, generating offspring with unique genetic makeups. Lastly, gene flow, the movement of genes into or out of a population due to migration or mating, introduces new genetic diversity, enhancing the variability within a population.
Unveiling Genetic Variation: The Fabric of Evolutionary Change
In the grand narrative of evolution, genetic variation plays the pivotal role of providing the raw material for natural selection. This diversity, the foundation of evolutionary processes, arises through a fascinating interplay of mutations, recombination, and gene flow.
Mutations: Unveiling Hidden Potential
Mutations, random changes in DNA, introduce novel genetic sequences. These alterations can disrupt or enhance gene function, leading to both positive and negative consequences. For instance, a mutation in the insulin receptor gene can disrupt glucose metabolism, potentially leading to diabetes. Conversely, mutations in the CFTR gene can restore normal lung function in individuals with cystic fibrosis.
Recombination: Reshuffling the Genetic Deck
Recombination, the exchange of genetic material between homologous chromosomes, provides another avenue for genetic variation. During meiosis, the cell division that produces gametes (eggs and sperm), chromosomes pair up and swap segments, creating new combinations of alleles. Recombination ensures that offspring inherit a unique blend of genes from both parents, increasing the diversity of the gene pool.
Gene Flow: The Interplay of Populations
Gene flow, the movement of genes between populations, further contributes to genetic variation. Migration, the movement of individuals between populations, introduces new alleles and alters the genetic composition of recipient populations. Gene flow can also occur through the exchange of gametes, such as via pollination in plants.
The interplay of mutations, recombination, and gene flow generates a vast reservoir of genetic variation within populations. This diversity provides the raw material for natural selection to act upon, driving the evolution of traits and the shaping of species over time.
The Role of Environmental Factors in Phenotypic Variation
The diversity of life on Earth is a testament to the incredible power of evolution. Natural selection, the driving force behind evolution, relies heavily on the variation within a species. This variation can be genetic, arising from mutations and other genetic changes, or phenotypic, which refers to the observable traits of an organism.
Phenotypic variation plays a crucial role in natural selection, as it provides the raw material for selection to act upon. However, phenotypic variation is not solely determined by genetics. Environmental factors also exert a significant influence on how organisms develop and express their traits.
Temperature and nutrition are two well-studied environmental factors that can affect phenotypic variation. For example, lizards living in warmer environments may have longer legs to facilitate faster movement, while those in cooler climates may have shorter legs to conserve heat. Similarly, plants grown in nutrient-rich soil may produce larger leaves, while those in nutrient-poor soil may exhibit smaller leaves with increased root development.
Other environmental factors, such as light and social interactions, can also influence phenotypic variation. Plants exposed to different wavelengths of light may alter their growth patterns, and animals living in social groups may develop behaviors that are influenced by the presence of others.
The impact of environmental factors on phenotypic variation is evident in the diversity of plant and animal populations across different habitats. For example, desert plants often have thick leaves and waxy cuticles to reduce water loss, while rainforest trees may have large, broad leaves to maximize sunlight absorption. Similarly, animals living in the Arctic may have thick fur and blubber to insulate against the cold, while those in the tropics may have lighter fur or feathers to facilitate heat dissipation.
Environmental factors do not directly change an organism’s genotype (genetic makeup). Instead, they interact with the genotype to influence the development and expression of traits. This interplay between genetics and environment is essential for understanding the complex mechanisms that drive phenotypic variation and the evolution of species.
Unveiling the Secrets of Inheritance: From Mendelian Laws to Non-Mendelian Mysteries
Mendelian Inheritance: Unveiling the Patterns
Gregor Mendel’s pioneering experiments in the mid-19th century laid the foundation for our understanding of inheritance. Through his meticulous work with pea plants, Mendel discovered that traits are inherited in a predictable manner. He proposed that each trait is controlled by two alleles, one inherited from each parent. Alleles can be dominant, meaning they are expressed even when only one copy is present, or recessive, requiring two copies to be expressed. The combination of alleles an individual inherits determines their phenotype, or observable traits.
Non-Mendelian Inheritance: Beyond Simple Patterns
However, nature often throws curveballs, and not all inheritance patterns conform to Mendel’s rules. Non-Mendelian inheritance describes the exceptions to the simple Mendelian patterns, introducing greater complexity to the world of genetics. Some non-Mendelian traits include incomplete dominance, where both alleles are expressed simultaneously, and co-dominance, where both alleles are fully expressed, resulting in a unique phenotype. Additionally, epigenetics plays a role in inheritance, influencing gene expression without altering the underlying DNA sequence. These modifications can be passed down to offspring, providing a fascinating example of how environmental factors can impact heritable traits.
The Importance of Inheritance in Natural Selection
Inheritance is a fundamental pillar of natural selection, the driving force behind evolution. It is through inheritance that traits that provide an advantage to an organism are passed on to their offspring. Over time, this accumulation of advantageous traits can lead to the emergence of new and distinct species. Without inheritance, evolution would be impossible, as traits could not be passed down and accumulated within a population.
The study of inheritance, both Mendelian and non-Mendelian, provides a deep understanding of the intricate tapestry of life. It unveils the mechanisms that shape the diversity and complexity of the natural world. From Mendel’s groundbreaking discoveries to the complex interplay of epigenetics, inheritance continues to fascinate scientists and inspire wonder in all who seek to unravel its secrets.
Epigenetics: The Silent Orchestrator of Trait Transmission
In the symphony of natural selection, inheritance plays a crucial role, and epigenetics is the enigmatic conductor that influences how traits are passed down through generations. Epigenetics refers to alterations in gene expression that do not involve changes to the underlying DNA sequence.
Imagine a piano with white and black keys, where genes represent the keys and the genome represents the piano itself. Epigenetic modifications, like placing small objects on the keys, can change the way the piano sounds without altering the keys. These modifications can influence which genes are expressed, and to what extent, leading to diverse phenotypic outcomes.
One common epigenetic mechanism is DNA methylation, which involves the addition of a methyl group to DNA. This can silence gene expression by preventing RNA polymerase from binding to the gene. Conversely, DNA demethylation can activate gene expression.
Epigenetic changes can occur in response to environmental cues or as a result of randomness. They can be temporary or stable, lasting across multiple generations. For example, exposure to toxins can cause epigenetic modifications that affect the health of an individual and their offspring.
These modifications can have profound implications for evolution. They provide a flexible mechanism for organisms to adapt to changing environments without altering their genetic code. Epigenetic changes can also contribute to phenotypic diversity within populations, increasing the raw material for natural selection to act upon.
By uncovering the secrets of epigenetics, we gain a deeper understanding of how traits are inherited and how organisms evolve over time. Epigenetics is a testament to the intricate dance between genetics and environment, constantly shaping the tapestry of life.
Inheritance: A Cornerstone of Natural Selection
In the tapestry of evolution, inheritance weaves the intricate threads that connect generations, shaping the destinies of species. It is through the transmission of genetic material that traits are passed from parents to offspring, providing the raw material for natural selection to work its magic.
Genetic Legacy: Passing on the Blueprint
At the heart of inheritance lies the enigmatic molecule called DNA, the blueprint that encodes the instructions for every living organism. Each individual inherits a unique combination of DNA from their parents, a genetic mosaic that determines their physical and behavioral characteristics. These inherited traits, known as phenotypes, can range from eye color and height to disease susceptibility and personality.
Natural Selection’s Ally
Inheritance plays a pivotal role in natural selection, the driving force behind the evolution of life. Natural selection favors individuals with traits that enhance their survival and reproductive success within a given environment. By inheriting advantageous traits from their parents, offspring gain a head start in the competition for resources, mates, and survival.
Preserving Beneficial Traits
The process of inheritance ensures that beneficial traits are not lost but instead perpetuated within a population. Over time, as advantageous traits accumulate through natural selection, populations become better adapted to their environmental challenges. This accumulation of favorable traits through inheritance is known as adaptive evolution.
Challenging Assumptions: Non-Mendelian and Epigenetic Influences
While inheritance often follows the classical principles of Mendelian genetics, there are exceptions to the rule. Non-Mendelian inheritance patterns, such as those involving multiple genes or environmental interactions, add complexity to the genetic canvas. Additionally, epigenetics, the study of heritable changes in gene activity that do not alter the DNA sequence, further underscores the intricate relationship between inheritance and the environment.
Defining Natural Selection: The Power That Shapes Life
In the tapestry of life, evolution weaves its intricate patterns through the relentless forces of natural selection. This fundamental mechanism drives the transformation of species over countless generations, shaping their traits and adaptations to the ever-changing world around them.
Natural selection is the process whereby organisms with traits that confer a selective advantage in their environment outcompete those without. Imagine a vast expanse of land teeming with rabbits. Some are born with slightly longer legs, giving them an edge in escaping swift predators. Over time, as these rabbits successfully reproduce and pass on their advantageous trait, the gene pool of the population gradually shifts in favor of the long-legged individuals. This subtle yet profound shift marks the inexorable march of natural selection.
On a larger scale, natural selection can orchestrate the emergence of entirely new species. As populations become geographically isolated or encounter unique environmental challenges, different selective pressures can arise. Over many generations, the accumulation of advantageous traits can lead to significant genetic divergence, eventually resulting in the formation of separate species that are unable to interbreed.
The power of natural selection is evident in the incredible diversity of life on Earth. From the intricate camouflage of moths blending into dappled bark to the sophisticated echolocation systems of bats, each species bears witness to the transformative power of this evolutionary force.
Natural Selection: Unveiling the Intricate Dance of Life
Understanding the Essential Parts of Natural Selection
Natural selection, the driving force behind evolution, is a complex process that shapes the diversity of life on Earth. To unravel its intricacies, we must first understand its essential components.
Variation: The Foundation of Evolution
Variation, the bedrock of evolution, arises from genetic and phenotypic differences among individuals. Genetic variation, the raw material for selection, stems from mutations, recombination, and gene flow. Phenotypic variation, influenced by environmental factors, encompasses the observable traits that make each individual unique.
The Power of Inheritance: Passing on Traits
Inheritance, the transmission of traits from one generation to the next, plays a pivotal role in natural selection. Mendelian inheritance, governed by dominant and recessive alleles, provides a framework for understanding how traits are inherited. Non-Mendelian inheritance, encompassing complex patterns such as polygenic traits and epigenetic modifications, further enriches our knowledge of trait transmission.
Selection: The Driving Force of Change
Natural selection, the engine of evolution, favors advantageous traits that enhance an individual’s survival and reproductive success. Selection pressures, imposed by the environment and competition, drive the evolution of traits that increase fitness. Artificial selection, practiced by humans, alters the genetic makeup of species for desired characteristics. Sexual selection, driven by mate choice, promotes traits that enhance reproductive opportunities.
Time: A Gradual Journey
Evolution unfolds over vast stretches of geological time. Speciation, the formation of new species, is a gradual process that occurs over many generations. Generation time, the time it takes for one generation to succeed the previous one, influences the rate of natural selection.
Natural selection is a multifaceted and awe-inspiring process that has shaped the tapestry of life on Earth. By understanding its essential components – variation, inheritance, and selection – and the role of time in evolutionary change, we gain a profound appreciation for the intricate dance of life that has produced the astonishing diversity that surrounds us.
Selection: The Driving Force of Change
In the realm of natural selection, the environment plays a pivotal role in shaping the survival and reproductive success of individuals and populations. These selection pressures act as the guiding force that drives the evolution of traits, favoring those that enhance an organism’s ability to adapt and thrive within its niche.
Natural selection operates through a relentless process of filtering and refinement. Individuals with favorable traits that increase their chances of survival and reproduction gain a reproductive edge over those with less advantageous traits. This differential survival and reproductive success leads to a gradual shift in the gene pool of the population. Over generations, the frequency of these beneficial traits increases, while the frequency of less favorable traits decreases.
The strength and direction of selection pressures can vary significantly. They can be directional, favoring one particular trait value, stabilizing, maintaining an optimal trait value, or disruptive, favoring extreme trait values over intermediate ones. These selection pressures can arise from a multitude of factors, including predation, competition for resources, mate choice, and environmental fluctuations.
For instance, in a population of lizards, individuals with darker skin may be favored by selection in an environment with high levels of solar radiation. This darker pigmentation provides enhanced protection from the harmful rays, increasing their survival rates and reproductive success. Over time, the frequency of the “darker skin” gene increases in the population, ultimately leading to the evolution of a population of lizards with predominantly darker skin tones.
Moreover, selection pressures can also drive the evolution of more complex traits, such as behaviors, physiological adaptations, and even social interactions. In cooperative species, for example, individuals that exhibit altruistic behaviors may have a higher likelihood of survival and reproductive success. This selfless behavior increases the chances of survival for other members of the group, thus benefitting the entire population and driving the evolution of altruism.
Discuss geological time and its implications for evolutionary processes.
Geological Time: The Tapestry of Life’s Journey
Journey Through Eons
Imagine a grand scroll, unfurling the story of life on Earth, starting billions of years ago. This is geological time, a vast canvas upon which the tapestry of evolution has been woven. It’s a timescale that humbles us, spanning epochs and eons, each marking a chapter in the history of life.
Layering of Earth’s History
Like the layers of an ancient rock formation, geological time is stratified into eras, periods, and epochs. Each layer represents a distinct era of Earth’s history, with its unique environmental conditions, flora, and fauna. These layers chronicle the evolution of life, from the earliest traces of single-celled organisms to the diversity we see today.
Imprints of Evolution
The fossils preserved in these layers are like snapshots, capturing the forms of past life. They document the origin and diversification of species, showing how organisms have adapted and evolved over time. For example, the fossil record shows the transition from fish to amphibians, reptiles to mammals.
Slow Yet Steady Transformation
Evolution, though gradual, is relentless, shaping species through a continuous process. The passage of geological time allows for the accumulation of genetic changes, and the forces of natural selection shape these changes into adaptive traits. Over eons, small changes can lead to profound transformations.
Geological time provides the context for understanding the history of life and the evolution of our planet. It reminds us of the immense timescale of evolution, a process that continues to unfold today. As we appreciate the grandeur of geological time, we gain a deeper understanding of our place in the interconnected web of life.
Evolutionary Time: The Grand Canvas of Life’s Transformation
As we navigate the vast expanse of natural selection, we come face-to-face with the remarkable yet patient hands of evolutionary time. Time, measured in geological epochs and generation intervals, orchestrates the grand ballet of speciation and divergence, the pivotal moments that sculpt the tapestry of life on Earth.
Speciation: The Genesis of New Species
Picture a sprawling landscape teeming with life. Within this vibrant tapestry, subtle variations accumulate over generations, shaping unique genetic profiles among individuals. These genetic differences can be as subtle as a shift in eye color or as profound as the development of new physiological adaptations.
As natural selection favors certain traits, these genetic variations become more prevalent within populations. Over time, the accumulation of such advantageous traits leads to the formation of isolated groups with distinct characteristics. These distinct populations, reproductively isolated from one another, embark on their own evolutionary trajectories, eventually blossoming into new, distinct species.
Divergence: The Symphony of Splitting Lineages
The evolutionary journey doesn’t end with speciation. The newly formed species continue to diverge as they navigate different environments and face unique selective pressures. These pressures mold their genetic makeup and phenotypic features, causing them to gradually drift apart.
The pace of divergence is influenced by various factors, including generation time, the rate at which populations reproduce and accumulate new genetic variation. Short generation times, as seen in rapidly reproducing organisms like bacteria, accelerate the process of divergence, while longer generation times, characteristic of large mammals, slow it down.
Through the relentless passage of evolutionary time, diverging species accumulate increasingly distinct genetic and phenotypic differences. They may develop new adaptations, lose ancestral traits, or evolve mating behaviors that prevent interbreeding with their progenitors. The symphony of divergence unfolds, producing a mesmerizing array of life forms that enrich our planet’s biodiversity.
The Role of Generation Time in Natural Selection
Generation time refers to the average time it takes for one generation of a species to replace the previous one. It is a crucial factor in natural selection as it determines the rate at which genetic changes can accumulate within a population. Shorter generation times allow for more rapid evolutionary change, while longer generation times result in slower evolutionary processes.
For instance, consider two species with different generation times: the annual plant Arabidopsis thaliana (generation time of about one year) and the Pacific Ocean sea turtle (generation time of about 30 years). In the case of Arabidopsis, natural selection can act swiftly on genetic variations that arise. In contrast, in the case of the sea turtle, due to its significantly longer generation time, evolutionary changes take place over a much longer period.
The length of generation time also influences the strength of selection pressure. Species with shorter generation times are more likely to experience the rapid effects of natural selection, as they can produce and evaluate many successive generations in a relatively short period. This allows natural selection to quickly weed out disadvantageous traits and promote the spread of beneficial traits.
On the other hand, species with longer generation times experience the effects of natural selection more gradually, as selection pressures take more time to shape the genetic makeup of the population. This can result in the persistence of less favorable traits and a slower overall rate of evolutionary change.
In summary, the generation time of a species plays a critical role in natural selection. Shorter generation times facilitate faster evolutionary change, while longer generation times lead to more gradual evolutionary processes. Understanding this relationship is essential for comprehending the dynamics of natural selection and the mechanisms of evolution.