Nadph: Fueling Photosynthesis Through Light-Driven Electron Transfer
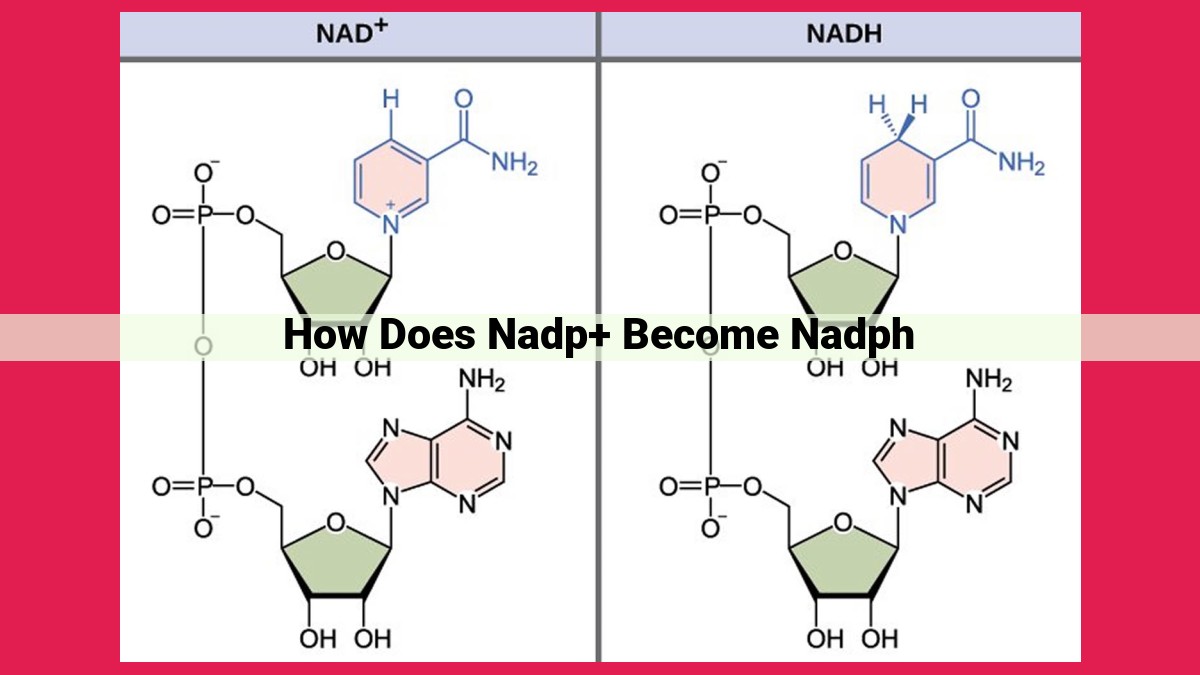
NADP+ is converted to NADPH through a series of reactions in the light-dependent stages of photosynthesis. Light energy absorbed by chlorophyll triggers water splitting, releasing electrons that ascend an electron transport chain, generating ATP. Electrons from PSI are transferred to ferredoxin, which then reduces NADP+ through NADP+ reductase. The resulting NADPH is the electron donor for the Calvin cycle, fueling carbon dioxide reduction.
Photosynthesis: Unveiling the Secret of Light Energy Conversion
Photosynthesis, the lifeblood of our planet, is a remarkable process that transforms light energy into chemical energy, fueling the growth and survival of all living organisms. At the heart of this process lies a crucial molecule: NADP+, the electron carrier that powers the light-dependent reactions.
The Light-Dependent Dance
Within the chloroplasts, the tiny organelles that house photosynthesis, light-dependent reactions unfold within the thylakoid membranes. Here, the green pigment chlorophyll captures light energy, orchestrating a cascade of events. Water molecules are split, releasing protons, electrons, and oxygen. These electrons embark on an intricate journey through an electron transport chain, a series of proteins that shuttle them from one complex to another.
As these electrons flow through the chain, they generate a proton gradient, a reservoir of chemical energy. This gradient drives the synthesis of ATP, the energy currency of the cell. Concurrently, electrons are transferred to ferredoxin, a protein that channels them to NADP+ reductase, an enzyme that ultimately reduces NADP+ to NADPH.
Light-dependent Reactions:
- Explanation of the reactions occurring in the thylakoid membranes of chloroplasts.
- Role of chlorophyll in absorbing light energy and splitting water molecules.
- Proton generation for ATP production and electron transfer.
Light-Dependent Reactions: Unraveling the Energy-Conversion Process
In the realm of photosynthesis, the light-dependent reactions take center stage, orchestrating a remarkable transformation from light energy to chemical energy. These reactions unfold within the thylakoid membranes of chloroplasts, the photosynthetic powerhouses of plant cells.
At the heart of this process lies chlorophyll, a remarkable pigment that absorbs light’s energy. This absorbed energy is harnessed to split water molecules, liberating electrons, protons, and oxygen. The protons are then pumped across the thylakoid membrane, creating an electrochemical gradient that drives the production of ATP, the cellular energy currency.
The electrons released from water embark on an energy-rich journey, passing through a series of protein complexes embedded in the thylakoid membrane. This electron transport chain resembles a relay race, with each protein complex passing the electron baton to the next, releasing energy that is captured as ATP.
The final destination for these electrons is the NADP+ reductase enzyme. Here, electrons are utilized to reduce NADP+ to NADPH, a crucial electron carrier in the Calvin cycle. NADPH provides the energy for carbon dioxide fixation, the process that converts inorganic CO2 into organic molecules.
Thus, the light-dependent reactions are the cornerstone of photosynthesis, harnessing light energy to produce ATP and NADPH, the essential building blocks for the plant kingdom’s life-giving processes.
Photosynthesis: The Energy Conversion Process: Unlocking the Secrets of Photosystem I and II
In the verdant world of photosynthesis, two enigmatic players take center stage: Photosystem I (PSI) and Photosystem II (PSII). These intricate molecular machines orchestrate a mesmerizing dance of electrons and energy, driving the conversion of sunlight into the chemical energy that sustains life on Earth.
The Symphony of PSII: Water Splitter and Electron Liberator
PSII, the maestro of the light-dependent reactions, resides in the thylakoid membranes of chloroplasts. Its mission is twofold: water splitting and electron release. With each beat, PSII harnesses the sun’s rays and uses them to tear apart water molecules. This process, known as photolysis, generates oxygen as a byproduct.
But PSII’s true artistry lies in its ability to liberate electrons. As the photons strike the chlorophyll molecules within PSII, they excite electrons, propelling them into a higher energy state. These energized electrons embark on a journey, leaving PSII poised to accept replacements.
PS(I) Shall Prevail: Electron Excitation and Ferredoxin Transfer
PSI, the second virtuoso in this photosynthetic ensemble, stands ready to receive the excited electrons from PSII. PSI also harbors chlorophyll molecules, which absorb photons to further excite the electrons. This surge of energy allows the electrons to escape from PSI’s grasp.
The vagabonds among the electrons now have a new destination: ferredoxin. This electron carrier shuttles them away from PSI, carrying them to the next stage of photosynthesis.
The Significance of NADP+ Reductase: Harnessing Electron Power
As the electrons journey through the electron transport chain, they encounter an indispensable enzyme: NADP+ reductase. This enzyme utilizes the electrons from ferredoxin to reduce NADP+ molecules. NADPH, the reduced form of NADP+, subsequently becomes a crucial electron carrier, powering the reactions of the Calvin cycle, where carbon dioxide is converted into life-giving glucose.
In this intricate photosynthetic symphony, PSI and PSII play distinct yet complementary roles. PSII sets the stage by splitting water and liberating electrons, while PSI elevates the electrons’ energy levels and channels them to ferredoxin. NADP+ reductase then steps in, capturing the electrons and transforming them into NADPH, the driving force behind the Calvin cycle.
NADP+ Reductase: The Unsung Hero in Photosynthesis
Photosynthesis, nature’s marvelous process, relies on light energy to transform carbon dioxide into nutrient-rich sugars. Within this complex ballet of reactions, NADP+ reductase plays a pivotal role, akin to a skilled conductor guiding electrons to their designated destination.
The Electron Carrier’s Journey
As light strikes chlorophyll molecules embedded within the chloroplast’s thylakoid membranes, it initiates a cascade of electron transfers. These electrons, liberated from water molecules by the splitters of Photosystem II, embark on an intricate journey through a series of electron carriers.
Among these carriers is the humble but mighty NADP+ reductase. This enzyme serves as the final stop in the light-dependent reactions, where it uses electrons carried by ferredoxin to reduce NADP+. This reaction, a chemical dance of sorts, yields NADPH, an electron-rich molecule that holds the key to unlocking the next stage of photosynthesis.
NADPH: The Lifeline of the Calvin Cycle
NADPH is not just any electron carrier; it’s the lifeline of the Calvin cycle, the metabolic pathway responsible for transforming carbon dioxide into sugars. Within this cycle, NADPH donates its precious electrons to reduce molecules, ultimately leading to the synthesis of glucose and other essential organic compounds.
Without NADP+ reductase and its ability to produce NADPH, the Calvin cycle would come to a grinding halt, and the very foundation of plant life would crumble. It is a testament to the enzyme’s critical role that it is found in all photosynthetic organisms, from the smallest algae to the towering giants of the forest.
A Harmonious Ensemble
In the symphony of photosynthesis, NADP+ reductase plays an essential part, working in tandem with other components to orchestrate the intricate dance of electron transfers. Like a skilled accompanist, it provides the rhythm and harmony that guide the flow of electrons, ultimately powering the conversion of light energy into the sustenance that sustains all life.
The Electron Transport Chain: A Vital Energy Generator in Photosynthesis
In the intricate dance of photosynthesis, the light-dependent reactions are a captivating spectacle of energy conversion. At the heart of this process lies the electron transport chain, a series of specialized proteins that orchestrates the flow of electrons from Photosystem II (PSII) to Photosystem I (PSI).
Electron Symphony
Imagine a grand orchestra, where each protein plays a distinct note, contributing to the harmonious electron symphony. These proteins, embedded within the thylakoid membranes, create a conductive highway for electrons to travel. As electrons traverse this chain, their energy is harnessed to do remarkable work.
ATP Generation: Energy Currency for the Cell
Crucially, the electron transport chain is not merely a conduit for electrons. As they cascade through the proteins, their energy is captured and used to generate ATP, the cellular currency of energy. This ATP acts as the power source for various cellular processes, including the Calvin cycle, where carbon dioxide is converted into glucose.
The Players of the Chain
Among the key players in the electron transport chain are cytochrome bc1 complex, cytochrome b6f complex, and ATP synthase. Each complex performs a specific task, ensuring the smooth transfer of electrons and the efficient generation of ATP.
Significance: From Light to Life
The electron transport chain stands as a testament to the intricate elegance of photosynthesis. By harnessing the energy of light, it produces electron carriers (NADPH) and ATP, which are the essential ingredients for the Calvin cycle, the heart of carbon dioxide fixation. Ultimately, the electron transport chain lays the foundation for life on Earth, providing the energy and building blocks for plant growth and, by extension, the entire food chain.
The Role of NADP+ in the Light-Dependent Reactions of Photosynthesis
Photosynthesis is the fundamental process by which plants and other organisms convert sunlight into chemical energy. At the heart of this process is the light-dependent reactions, which rely heavily on a molecule called NADP+. Without NADP+, the entire process of photosynthesis would grind to a halt.
Light-Dependent Reactions
The light-dependent reactions occur in the thylakoid membranes of chloroplasts. Chlorophyll, a green pigment, absorbs light energy and uses it to split water molecules. This process releases protons, electrons, and oxygen.
The electrons released from water splitting are then passed through a series of proteins called the electron transport chain. As the electrons move through this chain, they release energy that is used to produce ATP, the energy currency of the cell.
Photosystem I and II
The electron transport chain is made up of two photosystems, Photosystem II (PSII) and Photosystem I (PSI). PSII is responsible for splitting water molecules and releasing electrons, while PSI captures light energy and excites electrons.
The electrons excited by PSI are then transferred to a molecule called ferredoxin. Ferredoxin then transfers these electrons to NADP+ reductase, an enzyme that uses the electrons to reduce NADP+ to NADPH.
NADP+ Reductase
NADP+ reductase is a crucial enzyme in the light-dependent reactions of photosynthesis. It is responsible for converting NADP+ to NADPH, which is used as an electron carrier in the Calvin cycle, the second stage of photosynthesis where carbon dioxide is converted into glucose.
Related Concepts
- Chlorophyll: The green pigment that absorbs light energy and initiates the light-dependent reactions.
- Water splitting: The process by which water molecules are split into protons, electrons, and oxygen.
- Electron acceptors: Molecules that receive electrons from other molecules, such as ferredoxin and NADP+.
- Plastocyanin: A protein that transfers electrons between PSII and PSI.
The light-dependent reactions of photosynthesis are essential for the production of ATP and NADPH, two molecules that are critical for the Calvin cycle and the overall process of photosynthesis. NADP+ plays a key role in these reactions, and without it, photosynthesis would not be possible.