Unlocking The Molecular Interplay: A Comprehensive Guide To Molecule Relationships
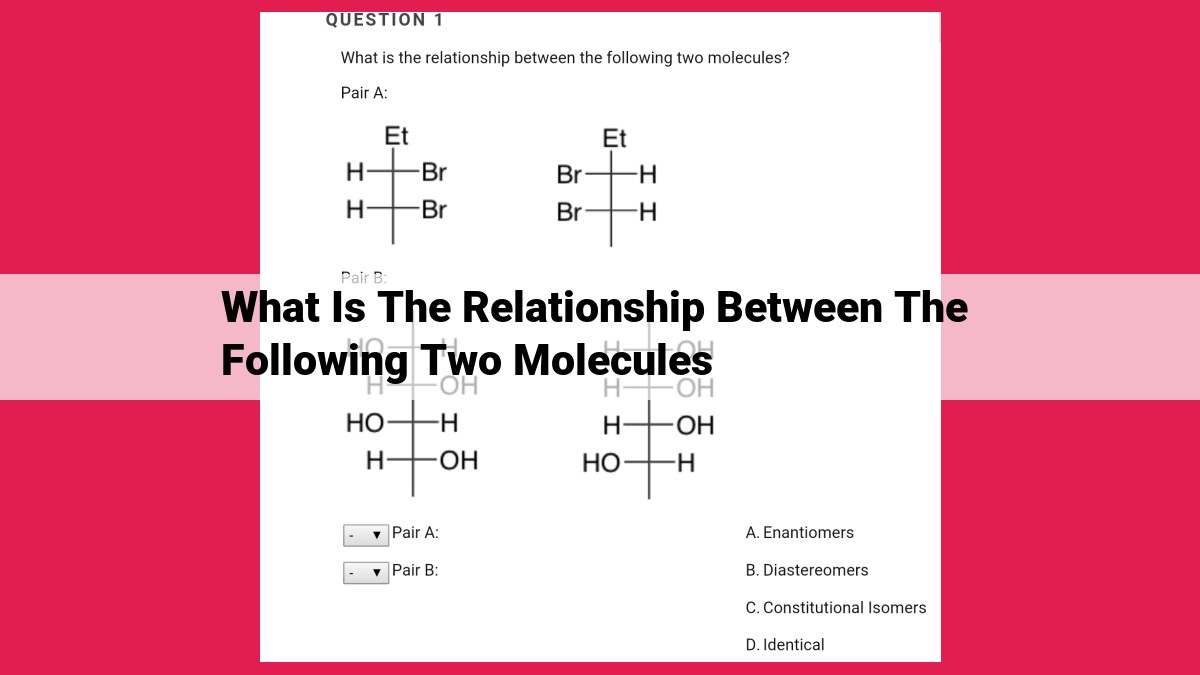
The relationship between molecules encompasses various aspects, including structural similarity, functional similarity, biosynthetic pathways, degradation mechanisms, binding affinity, evolutionary relationships, chemical reactivity, and physical properties. These aspects provide insights into molecular structure, function, interactions, and evolution, enabling a comprehensive understanding of biological systems. Understanding these relationships is essential for drug design, protein engineering, and unraveling complex biological processes.
Structural Similarity: Unveiling the Molecular Jigsaw Puzzle
In the intricate tapestry of life, molecules play a central role, their functions dictated by their unique structures. Structural similarity, the resemblance in the arrangement of atoms within molecules, serves as the cornerstone of understanding their relationships and predicting their behavior.
Like pieces of a puzzle, similar molecular structures often fit together to perform specific functions. Think of enzymes, the catalysts that power countless biological reactions. Enzymes exhibit structural similarity with their target molecules, allowing them to bind with precision and orchestrate chemical reactions with remarkable efficiency.
Structural similarity extends beyond enzyme-substrate interactions. Molecules with similar structures may also share similar functional similarities. For instance, molecules with analogous shapes may bind to the same receptor, triggering similar cellular responses. This principle enables researchers to predict the function of novel molecules based on their structural similarities to known compounds.
Moreover, structural similarity often reflects biosynthetic relatedness. Molecules synthesized through similar biochemical pathways tend to exhibit structural similarities. By analyzing these similarities, scientists can unravel the intricate biosynthetic networks that give rise to the vast diversity of molecules found in living organisms.
In the realm of molecular interactions, binding affinity plays a crucial role. The strength with which molecules bind to each other is influenced by their structural similarity. Molecules with complementary shapes exhibit higher binding affinities, enabling them to form stable complexes. This phenomenon underlies many biological processes, such as antibody-antigen interactions and protein-protein interactions.
Structural similarity is not merely a static property; it also provides insights into the evolutionary relationships between molecules. Similar molecular structures often indicate shared ancestry, as molecules diverge and adapt over time. Comparative analysis of molecular structures allows researchers to trace the evolutionary history of biological systems.
In conclusion, structural similarity is a fundamental concept in molecular biology, providing a framework for understanding molecular function, relationships, and evolution. By delving into the structural similarities between molecules, scientists gain invaluable insights into the complex interactions that govern the symphony of life.
Functional Similarity: Beyond Structural Resemblance
- Explore the concept of functional similarity and its implications for studying biological systems.
- Examine related concepts such as structural similarity, biosynthetic pathways, degradation mechanisms, and binding affinities.
Functional Similarity: The Hidden Orchestra of Biological Systems
In the intricate symphony of life, molecules play their assigned roles with precision, but some share an intriguing harmony that transcends their structural resemblance. Functional similarity unveils this hidden orchestra, revealing molecules that may look different but perform strikingly similar duties within biological systems.
Unveiling the Hidden Connections
Functional similarity arises when distinct molecules exhibit analogous biochemical functions, even if their physical structures differ. This shared choreography allows them to perform essential tasks within cells, tissues, and organisms. For instance, enzymes, the workhorses of metabolism, can have varying shapes yet catalyze the same chemical reaction, orchestrating vital metabolic processes.
Exploring Related Concepts
To fully grasp functional similarity, we must delve into its interconnected concepts:
- Structural similarity: While not always a prerequisite, similar structures often underlie functional similarity.
- Biosynthetic pathways: Molecules may share similar biochemical origins, indicating a common route of synthesis.
- Degradation mechanisms: Parallel degradation pathways can suggest functional redundancy or complementary roles.
- Binding affinities: Molecules with high affinity for the same ligands may perform related functions within biological networks.
Implications for Biological Studies
Understanding functional similarity has profound implications for our understanding of biological systems. By identifying molecules with shared functions, scientists can:
- Predict the functions of uncharacterized proteins or genes.
- Identify novel therapeutic targets for diseases associated with malfunctioning proteins.
- Gain insights into the evolution of biological systems and the emergence of new functions.
A Tapestry of Molecular Harmony
Functional similarity weaves a rich tapestry of interactions and dependencies within living organisms. It allows cells to adapt, respond to stimuli, and maintain homeostasis. As we delve deeper into this fascinating realm, we uncover the hidden connections that shape the delicate balance of life’s intricate symphony.
Biosynthesis: Unveiling the Molecular Symphony
In the intricate tapestry of life, the molecules that orchestrate our existence are not mere static entities but rather vibrant creations, forged in the fiery crucible of biosynthesis. This remarkable process, akin to a biological symphony, orchestrates the assembly of these molecular masterpieces, unveiling their structural and functional wonders.
Biosynthesis, the exquisite art of molecular construction, unfolds within the bustling metropolis of our cells. It involves a meticulously choreographed symphony of enzymes, each a master in its own craft, guiding the transformation of simple building blocks into intricate molecular structures. These molecular masterpieces, each with a distinct purpose, form the very fabric of life, defining our cellular machinery and orchestrating biological processes with unerring precision.
The intricate dance of biosynthesis is multifaceted, encompassing various pathways that lead to the creation of diverse molecules. Some pathways, like the symphony of amino acid synthesis, assemble the fundamental building blocks of proteins. Others, like the harmonious melodies of nucleotide biosynthesis, give rise to the genetic blueprints that guide our lives.
Beyond its pivotal role in crafting molecules, biosynthesis holds profound implications for understanding their structure and function. Molecules that share similar structural motifs often emerge from shared biosynthetic pathways, revealing a kinship that extends beyond mere appearance. Likewise, molecules with related functions may trace their lineage to common biosynthetic origins, suggesting a shared ancestry that has shaped their molecular destiny.
The tapestry of biosynthesis is intricately intertwined with other aspects of molecular life. It intertwines with degradation pathways, the molecular recycling system that dismantles molecules, and evolutionary relationships, the chronicle of molecular ancestry. Each thread in this intricate web of molecular interconnectedness tells a tale of life’s origin, function, and evolution.
By unraveling the secrets of biosynthesis, we gain not only a deeper understanding of the molecules that govern our existence but also a glimpse into the profound interconnectedness that weaves together the symphony of life.
Degradation: The Molecular Recycling System
In the bustling metropolis of cells, a continuous dance of creation and destruction unfolds. Molecules, the building blocks of life, undergo a life cycle that begins with synthesis and culminates in degradation. This essential process is like a cellular recycling system, breaking down molecules to release their constituent parts and repurpose them for new uses.
The Importance of Degradation
Degradation plays a pivotal role in cellular metabolism, the intricate network of chemical reactions that sustain life. By breaking down complex molecules, cells can generate energy, building blocks for new molecules, and eliminate waste products. Without degradation, cells would become overwhelmed with useless molecules, hampering their ability to function properly.
Mechanisms of Degradation
Degradation occurs through a variety of mechanisms, each tailored to specific types of molecules. Some molecules, like proteins, are broken down by enzymes called proteases. Other molecules, such as carbohydrates, undergo hydrolysis, a process that breaks them down using water. The resulting smaller molecules can then be recycled or excreted from the cell.
Related Concepts
Degradation is intricately linked to other molecular concepts:
- Structural Similarity: Molecules with similar structures often have similar degradation pathways.
- Functional Similarity: Molecules with similar functions may also share degradation mechanisms.
- Biosynthetic Pathways: The degradation pathways of molecules often mirror their biosynthetic pathways, the steps by which they were synthesized.
- Chemical Reactivity: The chemical reactivity of molecules can influence their susceptibility to degradation.
- Degradation Mechanisms: Different types of molecules have unique degradation mechanisms, tailored to their specific structures and functions.
Degradation is an indispensable process in cellular metabolism, ensuring the continuous turnover of molecules and the proper functioning of cells. By breaking down complex molecules, cells recycle essential components, generate energy, and remove waste products. The intricate mechanisms of degradation are a testament to the remarkable efficiency and adaptability of life’s molecular machinery.
Binding Affinity: The Key to Molecular Interactions
Introduction
In the intricate world of molecules, understanding their interactions holds immense significance for unraveling biological processes and driving innovation. Binding affinity, a fundamental concept in molecular biology, plays a pivotal role in these interactions, shaping the behavior of molecules and their impact on cellular function.
Defining Binding Affinity
Binding affinity is a quantitative measure that expresses the strength of the bond formed between two molecules. This strength is crucial as it determines the stability of the molecular complex and influences the rate and extent of reactions. Binding affinity is typically measured using techniques such as surface plasmon resonance and isothermal titration calorimetry.
Related Concepts
Binding affinity is closely intertwined with other molecular characteristics:
- Structural similarity: Molecules with similar structures often exhibit high binding affinity. The geometry and arrangement of functional groups contribute to the formation of complementary binding sites.
- Functional similarity: Molecules that share similar biological functions may have high binding affinity, as they are often involved in the same molecular pathways.
- Evolutionary relationships: Molecules that share evolutionary ancestry tend to have similar binding affinities, reflecting the preservation of binding sites and interactions over time.
- Binding mechanisms: Binding affinity is influenced by the types of chemical interactions involved, such as covalent bonds, hydrogen bonds, and electrostatic interactions. Understanding these mechanisms provides insights into the specificity and strength of molecular binding.
Importance in Molecular Interactions
Binding affinity governs numerous molecular interactions essential for life. Enzymes, for instance, rely on high binding affinity with their substrates to catalyze biochemical reactions. Ligand-receptor interactions enable the transmission of signals across cell membranes. Antibody-antigen binding is crucial for immune defense.
Applications in Drug Discovery
Understanding binding affinity is vital in drug discovery. Drugs are designed to bind specifically to target molecules with high affinity, ensuring their effectiveness and minimizing off-target effects. By manipulating binding affinity, researchers can optimize drug efficacy and reduce side effects.
Conclusion
Binding affinity is a fundamental aspect of molecular interactions, influencing the stability, specificity, and biological functions of molecules. Through its close relationship with structural similarity, functional similarity, evolutionary relationships, and binding mechanisms, binding affinity serves as a key determinant in understanding and manipulating molecular behavior. Its applications in drug discovery and beyond highlight its critical role in advancing scientific research and healthcare.
Evolutionary Relationship: Tracing the Molecular Ancestry
Unveiling the intricate tapestry of life’s diversity invites us on a captivating journey through evolutionary relationships. These relationships trace the lineage of molecules, connecting them across vast eons and providing insights into the history of life on Earth.
Structural Similarity: A Molecular Fingerprint
The structural similarity of molecules hints at their shared ancestry. Molecules with similar structures often share common building blocks and exhibit comparable shapes and arrangements. These similarities suggest a common progenitor, a molecule from which they evolved over time.
Functional Similarity: A Legacy of Ancestry
Beyond their structural resemblance, molecules may also possess functional similarity. They might perform similar tasks, offering clues to a shared evolutionary origin. For instance, enzymes with identical catalytic activities, despite differing structures, may have descended from a common ancestor.
Biosynthetic Pathways: A Chemical Genealogy
The biosynthetic pathways molecules take to form paint a vivid picture of their evolutionary relationships. Molecules that share similar pathways are likely to have originated from the same ancestral precursor. By tracing these pathways, scientists can reconstruct the evolutionary lineage of molecules.
Binding Affinities: The Dance of Interactions
The binding affinities of molecules, their propensity to interact with each other, provide additional clues about their evolutionary past. Similar binding affinities between different molecules suggest a common ancestor, as these affinities often reflect structural similarities inherited from their shared evolutionary origin.
Unraveling evolutionary relationships offers a profound understanding of molecular diversity. By exploring structural similarity, functional similarity, biosynthetic pathways, and binding affinities, we trace the molecular heritage of life on Earth. This knowledge not only deepens our appreciation for the interconnectedness of all living things but also empowers us to harness this evolutionary wisdom for advancements in biotechnology and medicine.
Chemical Reactivity: The Driving Force of Molecular Transformations
In the intriguing tapestry of life, where molecules dance and interact, a force governs their every move: chemical reactivity. It is the guiding principle that orchestrates molecular transformations, shaping their very essence and dictating their role in biological processes.
Chemical reactivity refers to the propensity of molecules to undergo chemical changes, forming or breaking bonds with other molecules. It determines how readily molecules interact, join forces, or break apart. This dynamic behavior underpins myriad biological events, from the metabolic breakdown of nutrients to the intricate signaling cascades that regulate cellular function.
Understanding chemical reactivity is thus essential for unraveling the mechanisms of life. It provides insights into the formation of complex biomolecules, such as proteins, lipids, and nucleic acids. It elucidates how enzymes catalyze biochemical reactions with astonishing precision and efficiency. Moreover, it sheds light on the degradation pathways responsible for recycling molecular components, ensuring cellular homeostasis.
Related Concepts Unraveled
Chemical reactivity is inextricably linked to several other molecular concepts. Degradation pathways, for instance, rely heavily on the chemical reactivity of molecules to break them down into smaller, reusable units. Physical properties, such as solubility, volatility, and ionization potential, also influence chemical reactivity and, consequently, molecular behavior.
By delving into the intricacies of chemical reactivity and its interconnectedness with other molecular concepts, scientists gain a deeper comprehension of the symphony of life. They can unravel the secrets of molecular transformations, decipher the language of biological processes, and harness the power of chemistry to improve human health and well-being.
Physical Properties: Shaping Molecular Behavior
Introduction:
In the captivating realm of molecular biology, the behavior of molecules holds immense significance. Beyond their structural and functional intricacies, their physical properties play a crucial role in shaping their interactions and biological impact.
Definition of Physical Properties:
Physical properties are measurable characteristics that describe the macroscopic properties of molecules without altering their chemical composition. They include attributes like solubility, density, melting point, boiling point, and refractive index.
Influence on Molecular Behavior:
These physical properties have a profound influence on molecular behavior in biological systems. For instance, solubility determines the ability of molecules to dissolve in different solvents, affecting their distribution and availability within cells and tissues. Melting point and boiling point govern the temperature at which molecules undergo phase transitions, influencing their stability and functionality under varying environmental conditions.
Chemical Reactivity:
Physical properties are also intimately linked to chemical reactivity. Molecules with high melting points tend to be less reactive due to their tightly packed structures. Conversely, molecules with low boiling points are more volatile and exhibit higher reactivity, facilitating interactions with other molecules.
Implications in Biological Systems:
The physical properties of molecules shape their behavior in various biological processes:
- Membrane Permeability: Molecules with low solubility in lipid-based membranes can struggle to cross cell membranes, influencing their cellular uptake and function.
- Protein Folding: The melting point of proteins is critical for their proper folding and stability, which is essential for their biological function.
- Drug Delivery: Modifying the solubility and boiling point of drugs can improve their delivery and efficacy in the body.
Conclusion:
Physical properties are essential factors in unraveling the complex behavior of molecules in biological systems. Understanding and manipulating these properties offer valuable insights into molecular interactions, disease mechanisms, and drug development strategies. By exploring the physical properties of molecules, scientists can gain deeper insights into the intricate tapestry of life and pave the way for groundbreaking advancements in healthcare and biotechnology.