Mole Ratio Of Hydrogen To Ammonia: Understanding Stoichiometry And Chemical Reactions
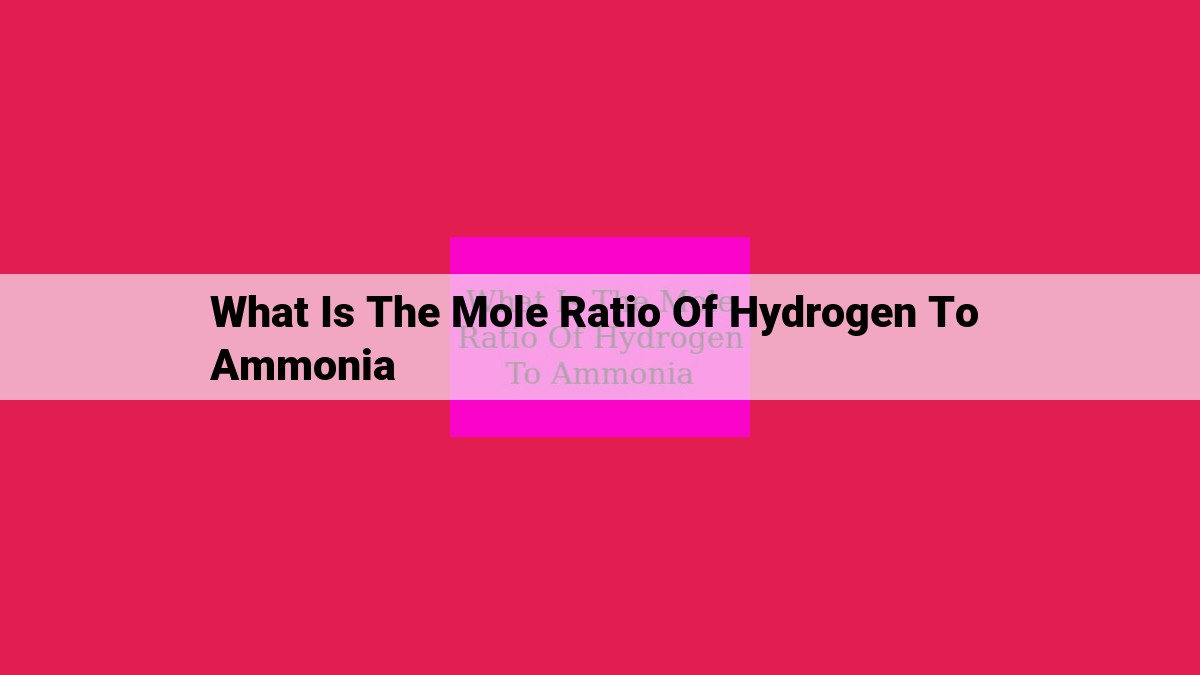
The mole ratio of hydrogen to ammonia is 1:2, as determined by the balanced chemical equation for the reaction between hydrogen and nitrogen to produce ammonia: H2 + N2 → 2NH3. This ratio signifies that for every 1 mole of hydrogen gas used in the reaction, 2 moles of ammonia are produced. It plays a crucial role in stoichiometry, allowing chemists to calculate the reactants and products required or formed in a chemical reaction based on the moles of any one of the substances involved.
Stoichiometry: Unraveling the Secrets of the Mole and Its Ratios
In the realm of chemistry, where the unseen world of atoms and molecules dance, understanding the mole and its ratios is paramount to grasping the intricate language of chemical reactions. The mole, a fundamental unit of measurement, quantifies the immense number of particles that constitute the building blocks of matter.
Stoichiometry, the backbone of chemical equations, shines a light on the precise relationships between reactants and products. It reveals the mole ratios that govern chemical reactions, enabling us to predict the quantities of substances involved and unravel the mysteries of chemical transformations.
Through this exploration, we’ll venture into the depths of the hydrogen-ammonia reaction, a prime example that unlocks the power of mole ratios.
Hydrogen: The Building Block of Matter
At the heart of chemistry lies the enigmatic element hydrogen, the firstborn among all elements and the lightest of all gases. It exists in its diatomic form, denoted as H2, where two hydrogen atoms forge an unbreakable bond.
As the genesis of the chemical tapestry, hydrogen plays a pivotal role in everything from the formation of stars to the sustenance of life on Earth. Its diatomic structure grants it unique properties, shaping its reactivity and bestowing upon it a volatile nature.
Hydrogen’s diatomic nature arises from its single valence electron, an electron eagerly seeking a dance partner. This dance often leads to the formation of covalent bonds with other atoms, creating a plethora of compounds that shape our world.
As the cornerstone of water, hydrogen enables the elixir of life to flow through our bodies and quench our thirst. It also forms the backbone of hydrocarbons, the fuel that powers our engines and industries.
Hydrogen’s diatomic nature not only influences its chemical behavior but also its physical properties. As a gas, H2 is colorless and odorless, making its presence known only through its interactions with other substances. Its low density allows it to float effortlessly, making it a promising candidate for lighter-than-air applications.
In the vast expanse of the cosmos, hydrogen reigns supreme. It forms the primordial clouds from which stars are born, and it fuels the nuclear reactions that power the Sun and other celestial bodies.
As we delve deeper into the realm of chemistry, we will uncover the intricate dance of hydrogen, exploring its diatomic nature and its fundamental role in shaping our universe.
Ammonia: A Versatile Chemical
In the realm of chemistry, ammonia stands out as an indispensable compound that finds applications in countless industries. Its colorless nature and pungent odor are instantly recognizable, but beneath its unassuming exterior lies a versatile chemical that plays a pivotal role in our daily lives.
Chemical Composition and Structure
Ammonia is a gas composed of nitrogen and hydrogen atoms. Its chemical formula, NH3, reflects the presence of one *nitrogen atom_ bonded to _three hydrogen atoms_. This unique structure gives ammonia its characteristic properties and reactivity.
Agricultural Significance
Ammonia’s most prominent application lies in the agricultural sector. It serves as a crucial component in the production of fertilizers, providing essential nitrogen to plants. Nitrogen is a macronutrient required for healthy plant growth, promoting lush foliage and increased yields. By utilizing ammonia as a source of nitrogen, farmers can enhance crop productivity and ensure food security for a growing global population.
Industrial Applications
Beyond agriculture, ammonia finds numerous uses in various industries. It is employed in the production of plastics, textiles, and pharmaceuticals. Additionally, ammonia is used as a cleaning agent and a refrigerant. Its ability to dissolve grease and remove stains makes it an effective cleaning solution, while its high heat capacity and low boiling point render it suitable for use in refrigeration systems.
Environmental Considerations
While ammonia offers substantial benefits, it is not without its environmental implications. Ammonia emissions can contribute to air pollution and acid rain. However, advances in production and handling techniques continue to mitigate these concerns. By adopting sustainable practices, industries can minimize the environmental footprint associated with ammonia production and utilization.
Chemical Equations: Unveiling the Language of Reactions
Chemical equations are the symbolic representations of chemical reactions—a powerful tool that allows us to decipher the intricate dance of elements and compounds as they transform into new substances. These equations serve as a shorthand, a visual language that captures the essence of chemical interactions.
At the heart of a chemical equation lies the concept of reactants and products. Reactants are the initial substances that undergo the transformation, while products are the newly formed substances that emerge from the reaction. These components are meticulously arranged on either side of an arrow, which symbolizes the direction of the reaction.
The placement of reactants and products is not merely arbitrary. Reactants occupy the left-hand side of the equation, while products reside on the right-hand side. This arrangement is a reflection of the flow of the reaction, where the reactants evolve into the products.
Consider the following equation:
2H2 + O2 → 2H2O
In this equation, two molecules of hydrogen (H2) and one molecule of oxygen (O2) react to form two molecules of water (H2O). The reactants, H2 and O2, are placed on the left-hand side, while the product, H2O, appears on the right-hand side.
Balancing Equations: Ensuring Equality
- Explain the importance of balancing chemical equations to have equal numbers of atoms on both sides.
- Highlight its significance for accurate mole ratio calculations.
Balancing Equations: The Key to Understanding Chemical Reactions
In the realm of chemistry, precision is paramount. One crucial aspect of this precision lies in balancing chemical equations, a process that ensures the harmonious coexistence of reactants and products. Balancing equations involves tweaking coefficients (numbers in front of chemical formulas) to achieve a state of equilibrium, where the number of atoms of each element remains the same on both sides of the equation.
The significance of balancing equations cannot be overstated. It serves as the foundation for accurate mole ratio calculations, which are essential for predicting the quantities of reactants and products involved in a chemical reaction. Without balance, mole ratios would be meaningless, leading to incorrect predictions and potential experimental mishaps.
Let’s delve into a real-world example to illustrate the importance of balancing equations. Consider the reaction between hydrogen and nitrogen gases to produce ammonia:
H2 + N2 → 2NH3
Initially, this equation is unbalanced, with 2 nitrogen atoms on the right side and only 1 on the left. To balance it, we adjust the coefficients as follows:
**3H2** + **N2** → **2NH3**
Now, the equation is balanced, with 3 atoms of hydrogen and 2 atoms of nitrogen on both sides. This balanced equation provides us with the crucial mole ratio of 3:1:2, indicating that for every 3 moles of hydrogen gas used, 1 mole of nitrogen gas is consumed and 2 moles of ammonia are produced.
Balancing chemical equations is a fundamental skill in chemistry. It not only ensures accuracy in mole ratio calculations but also allows us to predict reaction stoichiometry and product yields. By understanding the importance of balancing equations, we lay the groundwork for successful chemical reactions and pave the way for scientific advancements.
Mole Ratio in the Hydrogen-Ammonia Reaction: A Story of Stoichiometry
When it comes to chemistry, understanding the concept of moles is crucial. A mole represents a specific quantity of atoms or molecules, just like a dozen represents a specific quantity of eggs. And just as we use ratios to describe the relationship between different quantities (like eggs to dozen), in chemistry, we use mole ratios to describe the relationship between reactants and products in a chemical reaction.
Let’s take the production of ammonia as an example. Ammonia, a versatile chemical used in fertilizers, is produced through a reaction between hydrogen (H2) and nitrogen (N2). The balanced chemical equation for this reaction is:
*H2 + N2 → 2NH3*
This equation tells us that for every 1 mole of hydrogen gas that reacts, 2 moles of ammonia are produced. This relationship is known as the mole ratio between hydrogen and ammonia. It’s a crucial piece of information for chemists because it allows them to predict the amount of reactants and products involved in the reaction.
To determine the mole ratio, we simply look at the coefficients in front of each chemical formula in the balanced equation. In this case, the coefficient in front of hydrogen (H2) is 1, while the coefficient in front of ammonia (NH3) is 2. Therefore, the mole ratio of hydrogen to ammonia is 1:2.
This mole ratio has important implications for reaction stoichiometry. It tells us that for every 1 mole of hydrogen gas used, we need to have 1 mole of nitrogen gas and will produce 2 moles of ammonia. This knowledge is essential for chemists to ensure they have the correct proportions of reactants to achieve the desired yield of products.
In summary, the mole ratio in the hydrogen-ammonia reaction is 1:2, meaning for every 1 mole of hydrogen gas used, 2 moles of ammonia are produced. Understanding this mole ratio is fundamental for accurate mole calculations and predicting product yields in chemical reactions.
**Delving into the Mole Ratio: Unlocking the Secrets of Chemical Stoichiometry**
The Importance of the Mole Ratio in Chemical Equations
The mole ratio is a pivotal tool in chemistry that plays a crucial role in understanding the stoichiometry of chemical reactions. Stoichiometry refers to the precise numerical relationships between reactants and products in a chemical equation. The mole ratio ensures that these relationships are balanced, guaranteeing the conservation of mass and accurate predictions of product yields.
A Case Study: Hydrogen and Ammonia
Let’s delve into a specific example to illustrate the significance of the mole ratio. Consider the reaction between hydrogen gas (H2) and nitrogen gas (N2) to produce ammonia (NH3). The balanced chemical equation for this reaction is:
1 H2 + 1 N2 → 2 NH3
Decoding the Mole Ratio
The mole ratio is embedded within this balanced equation. The coefficients in front of each chemical formula represent the relative number of moles involved in the reaction. In this case, the equation indicates that:
- For every 1 mole of hydrogen gas used in the reaction, 2 moles of ammonia are produced.
This mole ratio has far-reaching implications for reaction stoichiometry and predicting product yields.
Implications for Stoichiometry and Product Yield
The mole ratio dictates the exact amount of reactants and products that will be involved in the reaction. This knowledge enables chemists to:
- Determine the limiting reactant, which is the reactant that runs out first and thus limits the amount of product formed.
- Calculate the theoretical yield, which is the maximum amount of product that can be produced under ideal conditions.
- Optimize reaction conditions to maximize product yield.
Storytelling Takeaway
Imagine a chemical reaction as a recipe. The mole ratio is akin to a set of precise instructions that ensure the right ingredients are used in the right proportions to create the desired product. By understanding and utilizing the mole ratio, chemists can wield the power of chemistry to create countless valuable substances that underpin our modern world.