Mitochondrial Cristae: Maximize Energy Generation Through Surface Area Expansion
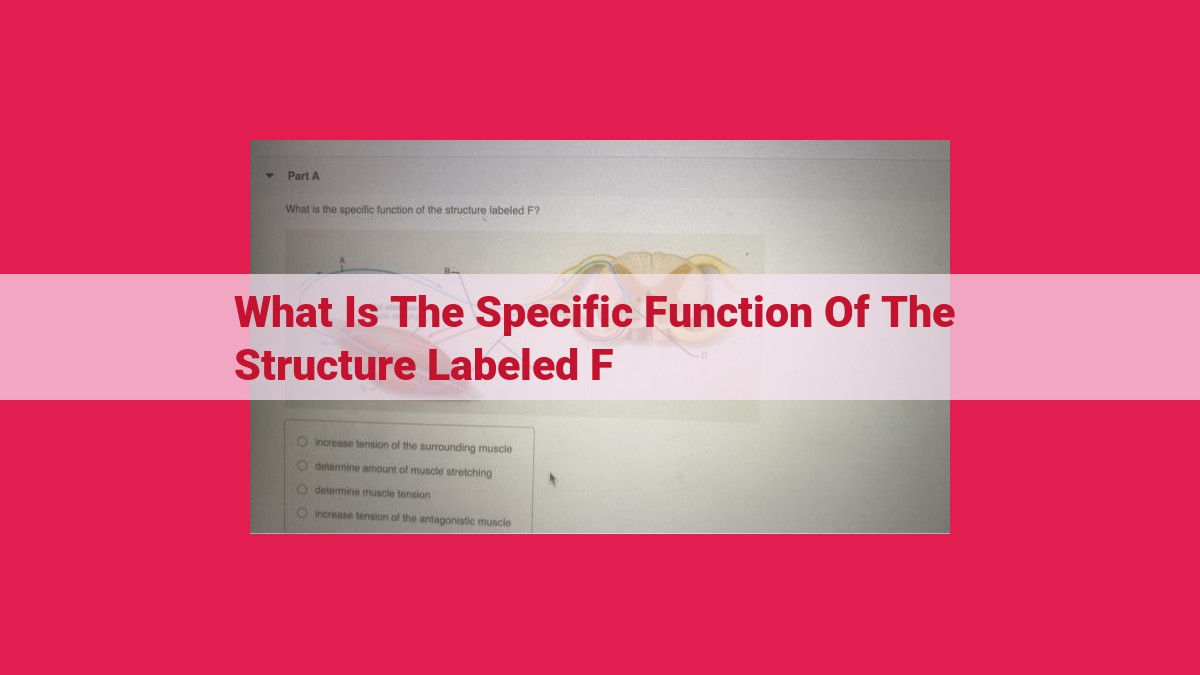
Structure f, known as cristae, are folded inner mitochondrial membranes that significantly increase the surface area. They are essential for housing proteins involved in the electron transport chain and oxidative phosphorylation, processes that drive cellular respiration and ATP production. The extensive cristae within mitochondria maximize energy generation and support the cell’s energy demands.
Understanding Mitochondria: The Powerhouses of Cellular Energy
In the vast kingdom of the cell, there exist enigmatic organelles that hold the key to our very existence: mitochondria. These cellular powerhouses are the unsung heroes responsible for our ability to breathe, move, and thrive.
Imagine mitochondria as miniature energy factories within each of our cells. They relentlessly produce adenosine triphosphate (ATP), the universal currency of energy for all life forms. Without mitochondria, our bodies would grind to a halt, leaving us lifeless and inert.
Their role in energy production is nothing short of miraculous. Mitochondria utilize a process called cellular respiration to convert nutrients like glucose into ATP. This intricate process involves the electron transport chain and oxidative phosphorylation, which generate most of the ATP our cells require.
One of the fascinating features of mitochondria is their double-membrane structure. This unique architecture creates a specialized environment within the organelle, allowing for efficient energy generation. The inner membrane is heavily folded into intricate structures called cristae, which dramatically increase the surface area for ATP production.
The Vital Importance of Cristae
Cristae are not mere folds; they are the powerhouses within the powerhouses. These structures are the sites of the electron transport chain, where high-energy electrons are transferred through a series of protein complexes. This process releases energy, which is harnessed to pump protons across the cristae’s membrane.
The proton gradient created by the electron transport chain drives oxidative phosphorylation, the final step in ATP production. Protons flow back through a channel, allowing protons to drive the synthesis of ATP from ADP.
In essence, mitochondria are the engines that drive our cells. They provide the energy that fuels our thoughts, movements, and every vital function that keeps us alive. Understanding these cellular powerhouses is essential for appreciating the intricacies of life itself.
Mitochondria: Powerhouses of the Cell
Imagine the bustling metropolis within your body, where trillions of tiny cells work tirelessly to keep you alive. At the heart of each cell lies a hidden power source, a tiny organelle known as the mitochondria. These cellular powerhouses are responsible for fueling your every action, from breathing to thinking.
Cellular respiration is the process by which glucose, a sugar molecule, is broken down to produce ATP, the energy currency of cells. This process takes place within the mitochondria. The mitochondria’s intricate inner membrane is folded into finger-like projections called cristae, which greatly increase its surface area. This increased surface area allows for more electron transport proteins to be embedded in the membrane, maximizing the potential for ATP production.
During cellular respiration, glucose is broken down in a series of steps, each releasing energy that is captured and stored in the bonds of ATP. ATP is then used as fuel by the cell’s various components, empowering everything from muscle contractions to protein synthesis. In this way, mitochondria serve as the energy factories of our cells, providing the fuel that powers our lives.
Unique Characteristics of Mitochondria: Unlocking the Powerhouse of the Cell
Mitochondria, the powerhouses of our cells, possess unique features that set them apart from other organelles. One of the most notable attributes of mitochondria is their double membrane structure: an outer membrane and an inner membrane enclosing a matrix-filled space. The outer membrane is relatively smooth, while the inner membrane is highly folded and contains cristae, which are shelf-like projections that provide a significant increase in surface area.
This increased surface area is not coincidental; it enhances the efficiency of cellular respiration, the crucial process by which mitochondria generate energy. The cristae contain protein complexes that participate in the electron transport chain, a series of reactions that generates the proton gradient necessary for oxidative phosphorylation (the synthesis of ATP). The more cristae there are, the greater the surface area for these reactions to occur, resulting in more efficient energy production.
Another distinctive characteristic of mitochondria is their unique DNA. Unlike the nuclear DNA found in the cell’s nucleus, mitochondrial DNA (mtDNA) is circular, smaller in size, and replicates independently. mtDNA carries genes crucial for mitochondrial function, particularly those involved in oxidative phosphorylation. Mutations in mtDNA can lead to various mitochondrial disorders that affect energy production and cellular health.
In essence, the double membrane structure, cristae, and unique DNA of mitochondria are key factors that enable these organelles to effectively generate ATP, fueling the countless cellular processes that sustain our bodies. By understanding these unique characteristics, we gain a deeper appreciation for the essential role mitochondria play in the symphony of life.
Cristae: The Powerhouse Within the Powerhouse
Delving into the Cellular Energy Matrix
Mitochondria, often referred to as the “powerhouses of the cell,” are remarkable organelles responsible for producing the energy that fuels our bodily functions. Within these tiny structures lies a complex network of structures known as cristae, playing a pivotal role in the energy-generating process.
The Inner Workings of Cristae
Cristae are intricate folds that extend into the mitochondrial matrix. Their primary purpose is to increase the surface area within the organelle, providing an extensive platform for crucial metabolic reactions. These folds are densely packed with proteins involved in the electron transport chain and oxidative phosphorylation, the two critical processes responsible for energy production.
The Electron Transport Chain: Powering the Mitochondria
The electron transport chain is a series of protein complexes located on the cristae membranes. Electrons, carrying chemical energy, pass through these complexes, releasing energy that is used to pump protons across the mitochondrial inner membrane. This proton gradient establishes an electrical potential, which drives the final step of energy generation: oxidative phosphorylation.
Oxidative Phosphorylation: Harnessing Energy from Protons
Through oxidative phosphorylation, the energy stored in the proton gradient is used to synthesize adenosine triphosphate (ATP), the universal energy currency of the cell. ATP is essential for powering all cellular processes, from muscle contraction to brain activity.
Cristae are fundamental to the energy-producing capabilities of mitochondria. By increasing the surface area within the organelle and facilitating the electron transport chain and oxidative phosphorylation, cristae ensure a continuous supply of ATP, the fuel that powers our very existence. Understanding these intricate cellular structures provides a deeper appreciation for the remarkable complexity and efficiency of the human body.
Mitochondria: Guardians of Cellular Fate in Apoptosis
Within the intricate tapestry of cellular life, mitochondria emerge as enigmatic organelles, holding the power to not only sustain but also extinguish the life of cells. In the realm of programmed cell death, known as apoptosis, mitochondria take center stage as pivotal players in initiating this essential process.
Apoptosis: The Orchestrated Dance of Cell Death**
Apoptosis is an orderly and controlled form of cell death that plays a crucial role in development, homeostasis, and disease. It is in these moments of sacrifice that mitochondria assume a dual role, both as sentinels detecting cellular distress and as orchestrators of the apoptotic cascade.
Guardians of the Threshold: Mitochondria as Sentinels
Mitochondria constantly monitor the cellular environment, sensing imbalances in energy production, oxidative stress, or DNA damage. When the threshold of tolerance is breached, mitochondria undergo subtle changes that initiate apoptosis. The key switch lies in the release of pro-apoptotic proteins, such as cytochrome c and Smac/DIABLO, from the intermembrane space into the cytosol.
The Unfolding Drama of Apoptosis
The release of these proteins into the cytosol sets off a chain reaction. Cytochrome c binds to Apaf-1, forming a complex that activates caspase-9, the initiator caspase. This event triggers a cascade of caspase activation, leading to the execution of the cell. Smac/DIABLO, on the other hand, neutralizes the inhibitory effect of XIAP (X-linked inhibitor of apoptosis protein), allowing the caspase cascade to proceed unimpeded.
The Significance of Mitochondrial Dysfunction in Disease
Mitochondria’s central role in apoptosis has profound implications for human health. Dysregulation of mitochondrial function can contribute to a wide range of diseases, including cancer, neurodegenerative disorders, and cardiovascular disease. Impaired mitochondrial function can lead to excessive apoptosis, contributing to tissue damage and organ failure. Conversely, suppressed apoptosis can result in uncontrolled cell growth and cancer.
Mitochondria, the cellular powerhouses, are not merely energy providers. They are also guardians of cellular fate, with the power to initiate the delicate dance of apoptosis when the cell’s integrity is compromised. Understanding the role of mitochondria in apoptosis is essential for unraveling the complex mechanisms of disease and developing therapeutic strategies to restore cellular balance and promote healthy living.