Millikan’s Oil-Drop Experiment: Unraveling Atomic Structure And Quantum Mechanics
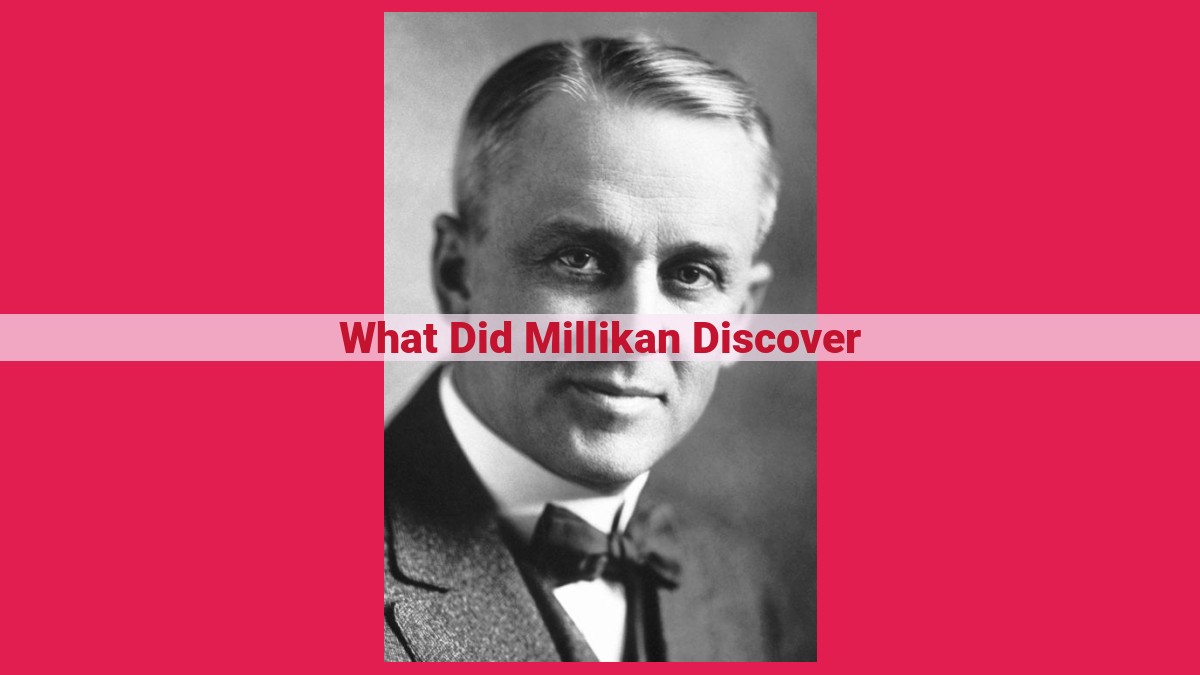
Through his groundbreaking oil-drop experiment, Millikan revolutionized understanding of atomic structure. He determined the charge-to-mass ratio of the electron, a pivotal discovery that unveiled the electron’s fundamental nature. This led to the establishment of the photoelectric effect, a phenomenon explained by Einstein’s equation that introduced the quantization of energy. Millikan’s work played a crucial role in the development of quantum mechanics, shaping our understanding of atoms, energy, and light.
Millikan’s Trailblazing Experiment: Unlocking the Secrets of Electrons
In the annals of scientific discovery, Robert Millikan’s oil-drop experiment stands as a seminal moment that revolutionized our understanding of atomic structure. This groundbreaking experiment, conducted in the early 20th century, provided irrefutable evidence for the existence of discrete units of charge, paving the way for the development of quantum mechanics.
Millikan’s experiment involved suspending tiny oil droplets in an electric field. By varying the strength of the field, he observed that the droplets acquired and lost charges in precise multiples of a fundamental unit. This observation led him to conclude that electrical charge is quantized, meaning it exists only in specific, discrete amounts.
The Millikan oil-drop experiment not only determined the charge of electrons, but also calculated their mass-to-charge ratio. This ratio became a crucial stepping stone in the development of quantum theory. By combining his results with Einstein’s theory of relativity, Millikan was able to determine the mass of electrons with unprecedented accuracy.
Millikan’s work had a profound impact on the scientific community, providing solid experimental support for the emerging theories of atomic structure. His oil-drop experiment remains a foundational experiment in physics, demonstrating the fundamental nature of electrical charge and the quantization of energy levels in atoms.
The Electron’s Charge-to-Mass Ratio: A Doorway to Quantum Phenomena
In the annals of scientific discovery, Robert Millikan’s oil-drop experiment stands as a pivotal moment, not just in the understanding of atomic structure but also as a gateway to the enigmatic world of quantum phenomena.
Millikan’s ingenious experiment utilized a clever apparatus that he designed himself. By suspending tiny oil droplets in an electric field, he meticulously measured their charge and mass. The precise control he achieved allowed him to determine a fundamental property of electrons: their charge-to-mass ratio.
This ratio proved to be a crucial stepping stone in advancing our understanding of atomic structure. By analyzing the behavior of charged oil droplets, Millikan gained insights into the nature of electrical forces within matter. His meticulous measurements ultimately led to the calculation of the electron’s charge and mass, providing scientists with a deeper understanding of the subatomic building blocks of the universe.
Millikan’s groundbreaking work not only illuminated the fundamental properties of electrons but also laid the groundwork for the development of quantum mechanics. His experiment served as a crucial bridge connecting the classical world of electricity and mechanics with the emerging realm of quantum physics. By uncovering the intricate relationship between charge and mass, Millikan paved the way for scientists to explore the quantum realm and unlock the secrets of atomic and subatomic particles.
The Photoelectric Effect: A Window into Albert Einstein’s Brilliance
In the early 20th century, a groundbreaking discovery shed light on the nature of light and laid the foundation for quantum mechanics. The photoelectric effect, observed when light strikes a material and causes the emission of electrons, defied classical physics and prompted Albert Einstein to propose a revolutionary theory.
Experimental Observations
When light strikes a metal surface, electrons can be ejected. Experiments revealed that the maximum kinetic energy of these emitted electrons is proportional to the frequency of the incident light. However, the intensity of light (number of photons) had no effect on the kinetic energy. This observation defied classical wave theory, which predicted that increasing the intensity would increase the electron’s energy.
Einstein’s Photoelectric Equation
To explain these puzzling observations, Einstein proposed a particle-like nature for light. He posited that light is made up of discrete packets of energy called photons. Each photon carries a specific quantum of energy, proportional to the light’s frequency.
Einstein’s photoelectric equation, E = hf, describes the relationship between the energy (E) of a photon, Planck’s constant (h), and the frequency (f) of the light. This equation suggests that each photon can transfer its entire energy to an electron.
Quantization of Energy and the Birth of Quantum Mechanics
The photoelectric effect provided strong evidence for the quantization of energy. The maximum kinetic energy of emitted electrons is the difference between the energy of the incident photon and the binding energy of the electron in the metal. This shows that energy can exist only in discrete, quantized units.
Einstein’s theory of the photoelectric effect revolutionized our understanding of light and matter. It laid the groundwork for quantum mechanics, the revolutionary theory that describes the behavior of subatomic particles and forms the basis of modern physics.
Planck’s Quantum Leap: Unraveling the Enigmatic Blackbody Radiation
In the realm of physics, the late 19th century marked a period of intense scientific inquiry. Physicists grappled with the paradoxical behavior of blackbody radiation, a phenomenon that defied explanation using the prevailing theories of classical physics.
The Enigma of Blackbody Radiation
A blackbody is an idealized object that absorbs all incident radiation, regardless of wavelength. When heated, blackbodies emit a continuous spectrum of light, with the intensity and wavelength distribution depending on the temperature. However, classical physics predicted a much higher intensity at shorter wavelengths than was observed experimentally. This discrepancy became known as the “ultraviolet catastrophe.”
Planck’s Breakthrough
Enter Max Planck, a brilliant German physicist. In 1900, Planck proposed a radical theory that revolutionized our understanding of blackbody radiation. He suggested that the energy emitted by blackbodies was quantized, meaning it existed in discrete, indivisible packets. Planck introduced a constant, now known as Planck’s constant, which represents the smallest possible unit of energy.
The Birth of Quantum Theory
Planck’s theory introduced a fundamental shift in physics. It showed that energy could not be continuously divided, but existed in discrete steps. This concept, known as quantization, laid the foundation for quantum theory, a new branch of physics that would profoundly impact our understanding of the atomic world.
Planck’s Legacy
Planck’s quantum theory not only solved the enigma of blackbody radiation but also laid the groundwork for quantum mechanics, one of the most successful and fundamental theories in modern science. Planck’s constant remains a crucial constant in the world of physics, appearing in countless equations that describe the behavior of subatomic particles and the interactions of light and matter.
Planck’s groundbreaking work earned him the Nobel Prize in Physics in 1918. His legacy continues to inspire generations of scientists, reminding us of the power of scientific exploration and the enduring impact of revolutionary ideas.
The Photon: A Particle of Light Unveiled
In the realm of quantum physics, where the macroscopic world gives way to the enigmatic subatomic realm, the discovery of the photon stands as a pivotal milestone. Robert Millikan’s groundbreaking work laid the foundation for this revelation, shedding light on the intricate nature of energy and the very essence of light itself.
Millikan’s meticulous experiments revealed that energy in atoms exists in discrete, quantized levels. This paradigm-shifting concept overturned classical physics’ notion of continuous energy and introduced the photon as the fundamental unit of light energy. Each photon carries a specific quantum of energy, directly proportional to its frequency.
The relationship between energy and wavelength is an intriguing aspect of the photon’s nature. As the energy of a photon increases, its wavelength decreases, while lower energy photons have longer wavelengths. This phenomenon manifests in the visible spectrum as a rainbow of colors, with high-energy blue light at one end and low-energy red light at the other.
The photon’s particle-like behavior has profound implications for our understanding of light. Once thought to be a continuous wave, light is now recognized as a stream of individual photons. This duality of light, both particle and wave, highlights the fascinating and often counterintuitive nature of the quantum realm.
The Proton: A Cornerstone of Atomic Structure
In the realm of atomic discovery, the proton emerged as a fundamental particle that laid the foundation for our understanding of the atomic nucleus and its structure. Its discovery and subsequent study by pioneering scientists, such as Ernest Rutherford, played a pivotal role in unraveling the secrets of the atom.
Protons: The Building Blocks of the Nucleus
The proton is a positively charged subatomic particle that resides in the heart of every atom, within the atomic nucleus. Along with neutrons, protons constitute the mass of the nucleus and define the identity of an element. The number of protons in an atom, known as its atomic number, determines the element to which it belongs. For instance, all atoms with one proton are identified as hydrogen, while those with two protons are classified as helium, and so on.
The concept of atomic number provides a crucial organizing principle in the Periodic Table of Elements. Elements are arranged in ascending order of their atomic numbers, reflecting the increasing number of protons in their nuclei. This arrangement unveils patterns and relationships that govern the chemical properties and behavior of elements, allowing scientists to predict and understand their interactions.
In conclusion, the discovery and understanding of the proton have been instrumental in shaping our knowledge of atomic structure and the very building blocks of matter. Its role in defining the identity of elements and organizing the Periodic Table has proven invaluable in advancing our comprehension of the chemical world.