Unlocking The Field Of View (Fov) In Microscopy: A Guide To Image Optimization
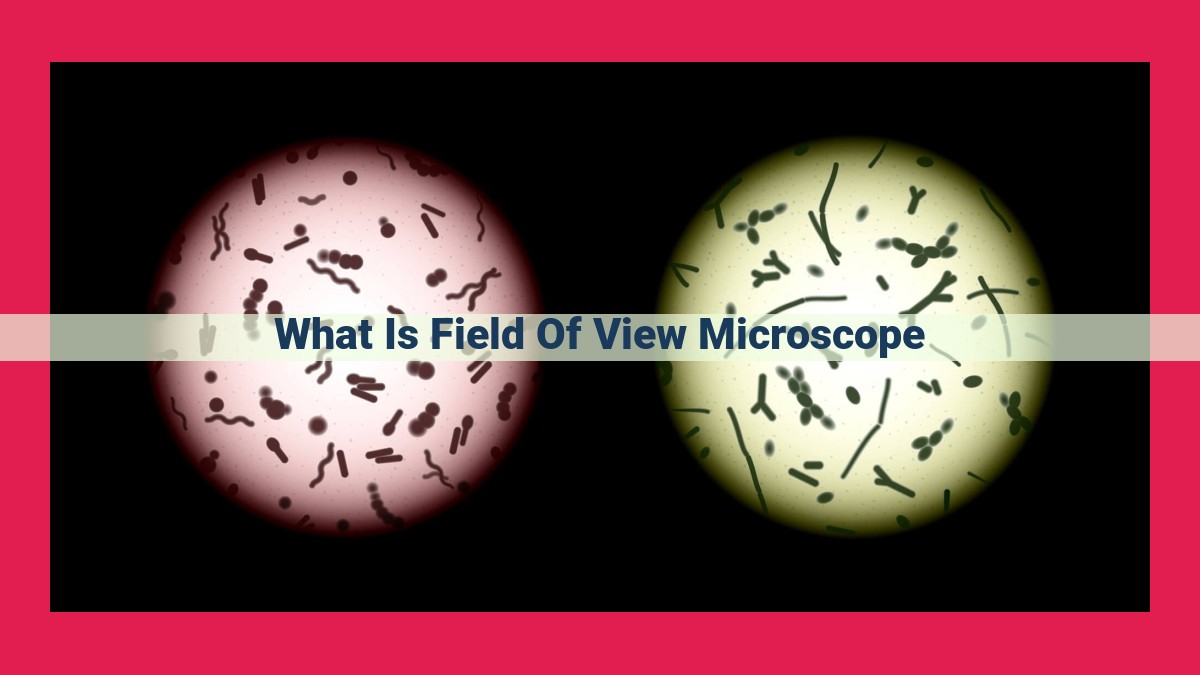
Field of View (FOV) in microscopy refers to the area of the specimen visible through the eyepiece or camera. It is influenced by factors like FOV number, diameter, and Numerical Aperture (NA). NA, which reflects the light-gathering ability of the objective lens, plays a crucial role in FOV, working distance, and image resolution. Optimizing the condenser’s position is essential for adequate illumination, while eyepiece magnification affects FOV and overall image size. Understanding FOV concepts is vital in selecting and using a microscope effectively for various applications.
Understanding Field of View (FOV) in Microscopy
Imagine yourself as a detective, meticulously examining a crime scene. Just as you need a clear view of the evidence, a microscopist requires a precise field of view (FOV) to effectively analyze microscopic specimens under the lens.
-
Definition of FOV: FOV refers to the circular area visible through the microscope’s eyepiece. Within this circular region, the specimen is magnified and illuminated, allowing the observer to examine its details.
-
Significance of FOV: The FOV is crucial in microscopy as it directly impacts the information and details that can be observed. A larger FOV allows for a wider view of the specimen, facilitating the identification and analysis of multiple structures or regions simultaneously.
-
Measuring FOV: FOV is typically measured in millimeters (mm) or micrometers (µm). The size of the FOV depends on several factors, including the magnification and numerical aperture (NA) of the objective lens, as well as the eyepiece being used.
Understanding these concepts and their relationships is essential for selecting the appropriate microscope and settings to meet specific research or diagnostic requirements.
Concepts Related to Field of View
Field of View (FOV) Number:
The FOV number indicates the linear diameter of the FOV in millimeters (mm), as viewed through the microscope at a specific magnification. A smaller FOV number corresponds to a higher magnification and a smaller area of the specimen that is visible.
Field of View Diameter:
The FOV diameter is the diameter of the circular area that is visible through the microscope. It is calculated by dividing the FOV number by the magnification. For instance, a microscope with a FOV number of 22 mm and a magnification of 100x would have a FOV diameter of 0.22 mm.
Numerical Aperture (NA):
Numerical Aperture (NA) is a crucial factor that influences the FOV. NA is a measure of the light-gathering ability of the objective lens. The higher the NA, the better the light-gathering capacity and the larger the FOV. NA also plays a role in depth of field, which is the range of distances in the specimen that appear in sharp focus.
Relationships Between FOV, NA, and Magnification:
The FOV is inversely proportional to both the magnification and the NA. This means that as magnification increases, the FOV decreases. Similarly, as NA increases, the FOV also decreases. This relationship is essential to consider when selecting the appropriate objective lens for a specific application.
Numerical Aperture (NA) and Its Impact on Microscopy
NA: The Keystone to Microscopy’s Powers
The world of microscopy is an intriguing realm where the magnification of lenses unveils the hidden wonders of the microscopic universe. However, there’s another crucial factor that plays a profound role in shaping our observations: Numerical Aperture (NA).
NA measures the light-gathering ability of a microscope objective, determining its capacity to capture fine details. A higher NA objective means greater light-gathering power and, consequently, a more detailed image.
NA’s Influence on Field of View
NA also significantly affects the field of view (FOV) of the microscope, which refers to the illuminated area visible through the eyepiece. A higher NA lens restricts the diameter of the illuminating cone of light, resulting in a narrower FOV. This may seem counterintuitive, but it’s essential for capturing sharper, more resolved images.
NA and Working Distance
NA is a balancing act that affects the microscope’s working distance. Working distance is the distance between the front lens of the objective and the specimen. A higher NA objective generally has a shorter working distance, requiring meticulous adjustment to prevent contact between the lens and the specimen.
Numerical Aperture, NA, like a skilled conductor leading an orchestra, harmonizes the elements of microscopy. It determines the light-gathering ability, field of view, and working distance, allowing us to tailor the microscope to our specific needs. Understanding NA empowers us to navigate the world of microscopy with precision, unlocking the secrets of the invisible realm.
Working Distance and Condenser
The working distance is the distance between the objective lens and the specimen. It’s an important factor to consider when choosing a microscope, as it determines how close you can get to the specimen without the lens touching it. A larger working distance allows you to examine thicker specimens or use specialized techniques like epifluorescence.
The working distance is closely related to the numerical aperture (NA). The higher the NA, the shorter the working distance. This is because a higher NA means the objective lens is collecting more light from a wider angle, which requires it to be closer to the specimen.
The condenser is an optical element that helps to focus light onto the specimen. It’s located below the stage and works in conjunction with the objective lens to create a bright, well-illuminated image. The condenser should be adjusted to match the numerical aperture of the objective lens. Using a condenser that is too small or too large can result in a loss of image quality.
By understanding the relationship between working distance, NA, and the role of the condenser, you can optimize the illumination of your specimens and achieve the best possible image quality.
Magnification and Eyepiece: Unveiling the Dynamics of Field of View
The field of view (FOV) in microscopy is like the window through which we observe the microscopic world. Magnification, the process of enlarging an image, plays a pivotal role in determining the size of this window. The higher the magnification, the closer we can zoom in on the specimen, but the smaller the FOV becomes. This is because as the magnification increases, the light from the specimen is spread over a smaller area in the image, resulting in a narrower FOV.
Eyepieces, also known as ocular lenses, are the lenses that we look through when using a microscope. They determine the final magnification of the image and, consequently, the FOV. Different eyepieces have different magnification powers, ranging from 5x to 20x or higher. The magnification power of an eyepiece is typically engraved on its side.
For instance, if we use a 10x eyepiece with a 10x objective lens, the total magnification will be 100x (10x eyepiece x 10x objective). This will provide a smaller FOV than using a 5x eyepiece with the same objective lens, which would yield a total magnification of 50x and a larger FOV.
Understanding the relationship between magnification, eyepieces, and FOV is crucial for optimizing the visualization of specimens under the microscope. By selecting the appropriate eyepiece and magnification, we can fine-tune the FOV to achieve the best balance between detail and overall view of the specimen.