The Science Behind Metal Colors: Understanding Optical Properties For Engineering, Art, And Architecture
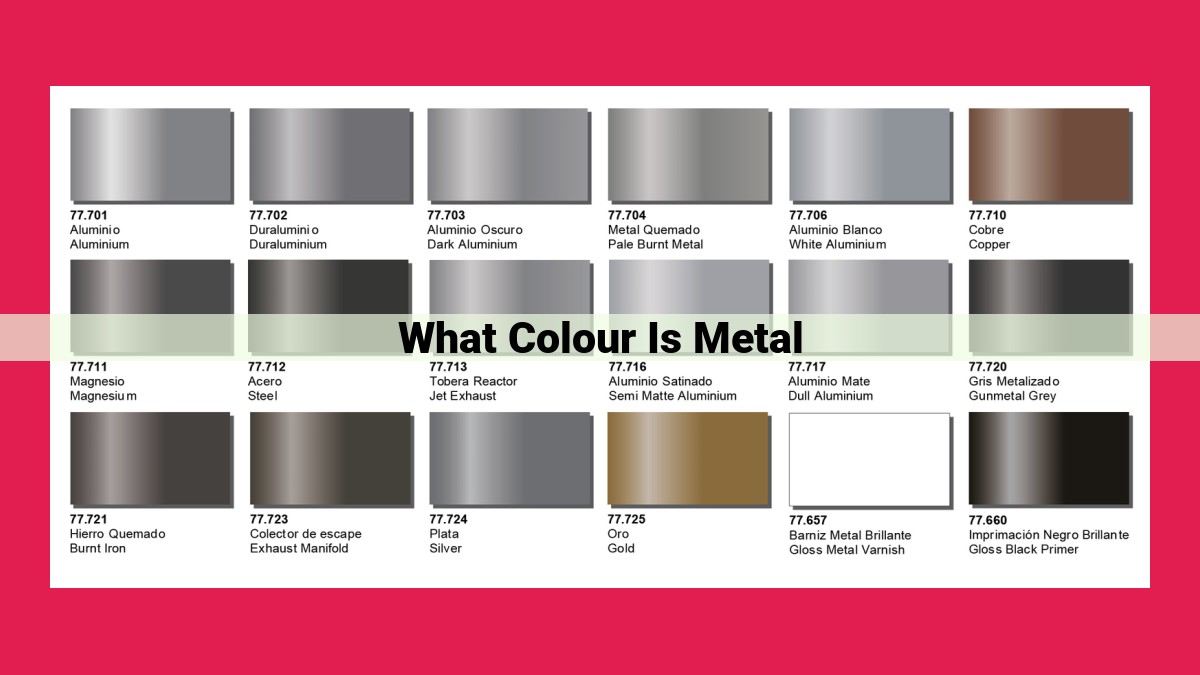
The color of metals is influenced by various factors, including metal oxides formed on their surfaces that alter their optical properties. Transition metals exhibit varying colors due to the electronic transitions of d-orbital electrons. Alloys, formed by combining different metals, can possess unique colors by altering the electronic structure. Specific surface treatments, such as anodizing, create colored oxide layers. Additionally, nanostructures display distinctive optical properties that affect their color. Understanding these mechanisms is crucial for applications involving color in engineering, architecture, and art.
Why Do Metals Change Color? Unraveling the Secrets of Metal Hues
We often take for granted the vibrant colors that metals showcase around us, from the shimmering gold of jewelry to the sleek black of cast iron cookware. But have you ever wondered what gives metals their distinctive hues? In this blog post, we’ll embark on a journey to uncover the fascinating world of metal colors, exploring the role of metal oxides and their impact on the visual appearance of these versatile materials.
Metal Oxides: The Hidden Colorists
When metals react with oxygen, they form metal oxides, compounds that play a crucial role in determining the color of the resulting material. The formation of metal oxides is a complex process involving the loss of electrons from the metal atoms, leading to the creation of positively charged metal ions and negatively charged oxide ions. These ions then combine to form metal oxides, with the specific properties of the oxide depending on the particular metal involved.
Color Symphony: The Palette of Metal Oxides
The color of metal oxides is largely influenced by the electronic structure of the metal ions. Metals with unfilled d-orbitals tend to form oxides with intense colors, as the d-electrons can absorb and re-emit light in a specific wavelength, giving rise to the observed color. For instance, iron(III) oxide (Fe2O3), commonly known as rust, appears red-orange due to the presence of unfilled d-orbitals in the iron ions. Conversely, metals with filled d-orbitals typically form colorless or white oxides, as the d-electrons are less likely to interact with light.
Metal oxides play a vital role in determining the color of metals. By understanding the formation and electronic structure of these compounds, we can appreciate the diverse hues that metals can exhibit. This knowledge not only enhances our understanding of materials but also opens up avenues for tailoring the color of metals for specific applications in engineering, architecture, and art. From the gleaming skyscrapers to the intricate jewelry we adorn, metal colors add a touch of beauty and intrigue to our world, reminding us of the hidden wonders that lie within the realm of chemistry.
The Kaleidoscopic Colors of Transition Metals
Embark on a fascinating journey into the vibrant realm of transition metals, where the interplay of electrons orchestrates a mesmerizing symphony of colors. At the heart of this chromatic dance lies a fundamental concept: d-orbital electrons.
D-orbitals are energy levels that can accommodate up to 10 electrons. When these levels contain electrons, they interact with photons of light to create distinctive colors. This captivating display, known as d-d transitions, grants transition metals their characteristic hues.
Take iron as an example. Its d-orbital electrons absorb blue and green wavelengths, leaving behind the crimson shade we associate with rust. In contrast, copper owes its warm, golden glow to the selective absorption of blue light. The iridescent blues and greens of titanium arise from the fine-tuning of its d-orbital electron configuration.
As we traverse the periodic table, we encounter a kaleidoscope of transition metals, each showcasing its unique chromatic identity. They’re the maestros behind the vibrant hues that adorn our world, from copper’s antique patina to gold’s regal luster and chromium’s silvery sheen.
Alloys: Transforming the Color of Metals
In the realm of metals, where countless hues dance before our eyes, we uncover the captivating world of alloys – a testament to human ingenuity and nature’s artistry.
An alloy is a magical blend, a harmonious fusion of two or more metals that creates a new substance with properties that transcend those of its individual components. Through this transformative process, metals acquire a breathtaking array of colors, from the vibrant gleam of rose gold to the enchanting brilliance of brass.
The secret behind this chromatic metamorphosis lies in the atomic dance of electrons. When different metals unite, their electrons engage in a lively exchange. This delicate interplay alters the electronic structure of the resulting alloy, influencing the way it interacts with light.
By carefully selecting the metals and their proportions, metallurgists can orchestrate the absorption and reflection of specific wavelengths of light. This symphony of light and matter gives rise to the mesmerizing hues that grace our jewelry, utensils, and countless other objects of everyday life.
For instance, the addition of copper to gold lends it a rosy flush, while the embrace of zinc transforms it into the warm glow of brass. These alloys, like alchemists’ potions, transmute the colors of their parent metals into something altogether captivating.
So, next time you marvel at the vibrant colors of the world around you, remember the enchanting power of alloys – a testament to the intricate dance of science, art, and human ingenuity. They remind us that even in the most mundane of materials, beauty and wonder await those who seek it.
Surface Treatments: Enhancing the Color Palette of Metals
When it comes to manipulating the color of metals, surface treatments emerge as a transformative force. These techniques empower us to alter the electronic structure and optical properties of metal surfaces, unlocking a vibrant spectrum of colors.
One seminal surface treatment is anodizing, particularly prevalent in the realm of aluminum. This electrochemical process harnesses the principles of oxidation to create a protective oxide layer on the metal’s exterior. The thickness and composition of this layer dictate the specific color imparted to the metal.
Anodizing allows for a breathtaking array of hues. For instance, porous anodic oxide films can scatter light, resulting in an iridescent display of colors. By precisely controlling the voltage, temperature, and electrolyte used in the anodizing process, manufacturers can achieve a spectrum of colors, ranging from subtle pastels to vibrant metallics.
Furthermore, anodizing not only enhances the aesthetic appeal of metals but also improves their durability. The protective oxide layer guards against corrosion and wear, making anodized metals ideal for applications in automotive, construction, and consumer electronics.
In essence, surface treatments are a testament to the malleable nature of metals. By manipulating their surfaces, we can unlock a world of colors and enhance their functionality. These techniques have revolutionized the way we interact with metals, enabling us to create visually stunning and practically robust materials.
Nanostructures: The Colorful World of Tiny Structures
In the realm of materials science, nanostructures hold a special place as they exhibit extraordinary optical properties that defy their diminutive size. These structures, measuring in nanometers (billionths of a meter), possess the unique ability to manipulate light in ways that conventional materials cannot.
Unique Optical Properties of Nanostructures
Nanostructures are characterized by a high surface area-to-volume ratio, which introduces a wealth of surface states that can interact with light. These surface states give rise to unique optical phenomena, including:
- Surface Plasmons: Surface plasmons are waves of oscillating electrons that propagate along the surface of nanostructures. They enhance light absorption and scattering, creating vibrant colors.
Plasmonic Effects
Plasmonic effects are responsible for the brilliant colors observed in many nanostructured materials. By controlling the size, shape, and composition of nanostructures, scientists can tune their plasmonic properties and produce a wide range of colors.
- Light Absorption and Scattering: Plasmons interact with incident light, selectively absorbing and scattering specific wavelengths. This selective interaction results in the appearance of characteristic colors.
Applications of Nanostructures
The unique optical properties of nanostructures have found applications in a variety of fields, including:
-
Color-Tunable Materials: Nanostructures can be tailored to create vibrant and customizable colors for use in pigments, dyes, and other optical components.
-
Sensor Technology: Nanostructured surfaces can be used to detect specific molecules or substances by monitoring changes in their optical properties.
-
Biomedical Imaging: Gold and silver nanostructures have been used as contrast agents to enhance the visibility of biological structures in imaging techniques.
In conclusion, nanostructures offer a fascinating and versatile platform for controlling and manipulating the color of materials. By understanding the unique optical properties of these tiny structures, scientists and engineers can create materials with tailored optical properties for a wide range of applications.