How To Measure Pressure Using A Manometer: A Step-By-Step Guide
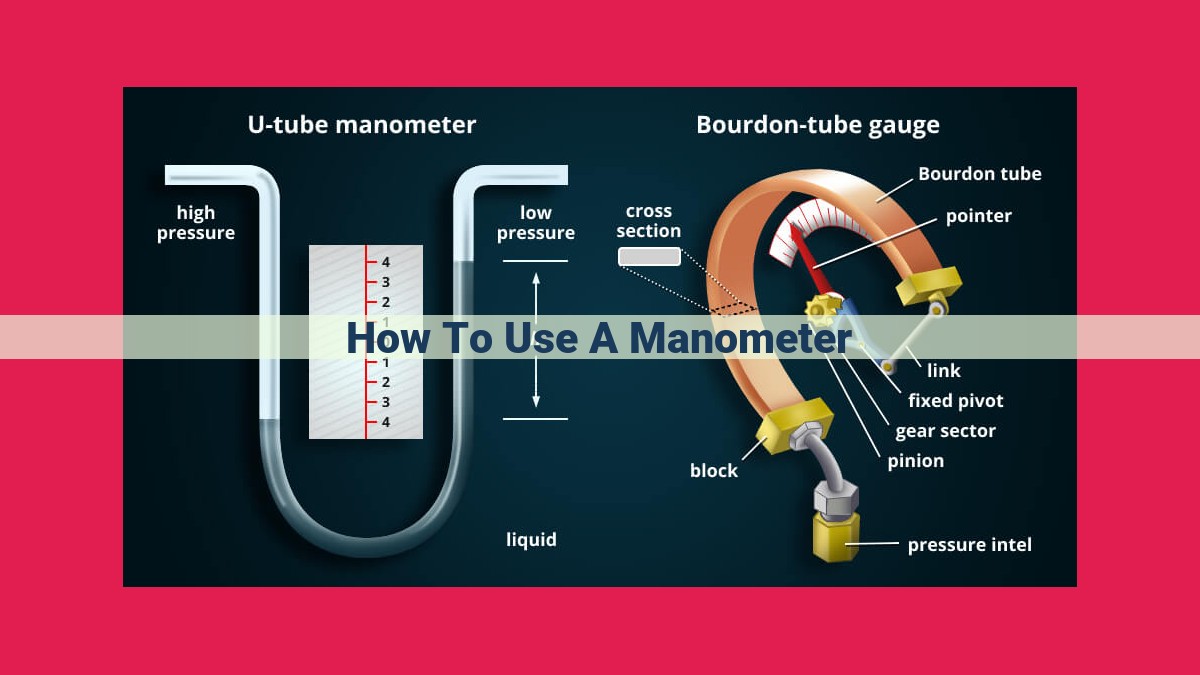
- Connect the manometer to the pressure source.
- Open the valve to allow the fluid to flow into the manometer.
- Read the difference in fluid levels between the two columns.
- Multiply the difference in fluid levels by the fluid density to get the pressure reading.
Navigating the World of Manometers: A Comprehensive Guide
In the realm of pressure measurement, manometers reign supreme. These versatile instruments empower engineers, technicians, and scientists to accurately gauge the pressing forces that shape our world. In this guide, we’ll delve into the fascinating world of manometers, unraveling their types, unraveling their applications, and unlocking the secrets of precise pressure measurement.
Chapter I: Types of Manometers – A Symphony of Design
Manometers come in all shapes and sizes, each tailored to specific measurement needs. Let’s explore the most common types:
-
Vertical Manometers: The classic silhouette, vertical manometers consist of a vertical glass tube and a fluid column, with pressure applied at the base. They offer simplicity and reliability, often used in low-pressure applications.
-
Inclined Manometers: A step up from their vertical counterparts, inclined manometers boast inclined tubes. Their sloping design amplifies pressure differences, resulting in greater sensitivity.
-
U-Tube Manometers: Picture an inverted U-shape; that’s a U-tube manometer! With a fluid-filled U-shaped tube, pressure is applied to one arm, displacing the fluid column. They’re ideal for measuring differential pressure.
-
Digital Manometers: The modern marvel, digital manometers utilize electronic sensors to measure pressure. They offer real-time readings, data logging, and a host of advanced features.
Chapter II: Measurement Types – Unraveling the Pressure Universe
Manometers unlock a world of pressure measurements:
-
Differential Pressure: The pressure difference between two points. Imagine a U-tube manometer; it captures this pressure gap.
-
Gauge Pressure: The pressure relative to the surrounding atmosphere. It’s the pressure we commonly encounter in our daily lives, measured against the ambient air pressure.
-
Absolute Pressure: The total pressure, including atmospheric pressure. This parameter reigns supreme in scientific and engineering applications.
Chapter III: Fluid Selection – The Liquid Symphony
The choice of fluid in a manometer is crucial:
-
Mercury: The traditional choice, mercury boasts high density for precise measurements. However, its toxicity concerns spark caution in modern applications.
-
Water: A readily available and non-toxic alternative, water’s lower density limits its sensitivity. Yet, it remains a reliable choice for low-pressure measurements.
-
Other Liquids: Specific applications demand specialized fluids. Oils, alcohol, and even refrigerants find their place in manometers, catering to unique measurement needs.
Measurement Types (Heading)
- Define differential pressure, gauge pressure, and absolute pressure.
- Explain how manometers measure each type of pressure.
Measurement Types in Manometers: Unraveling the Pressure Enigma
In the realm of pressure measurement, manometers stand as versatile tools, capable of quantifying the invisible force that governs our surroundings. To fully appreciate their capabilities, it’s essential to delve into the different measurement types they employ.
Differential Pressure: Unveiling the Pressure Difference
Imagine a scenario where you have two distinct pressure sources, such as a pressurized tank and an open atmosphere. The difference between these pressures is known as differential pressure. Manometers excel at measuring differential pressure by connecting to both sources and indicating the pressure disparity.
Gauge Pressure: Measuring Pressure Relative to the Environment
When you engage in everyday activities like inflating a tire or checking blood pressure, you’re dealing with gauge pressure. This type of pressure measures the difference between the actual pressure and the surrounding atmospheric pressure. Manometers employ a reference point at atmospheric pressure to accurately display gauge pressure.
Absolute Pressure: Capturing the Whole Pressure Picture
Absolute pressure takes a comprehensive approach by factoring in both gauge pressure and atmospheric pressure. It paints a complete picture of the pressure, providing a reference point of zero pressure in a vacuum. Manometers can be calibrated to measure absolute pressure by incorporating atmospheric pressure measurements.
Unveiling the Inner Workings of Manometers
To understand how manometers measure these diverse pressure types, it’s helpful to visualize a U-tube manometer. This simple device consists of a liquid-filled U-shaped tube connected to the pressure sources. The liquid levels in each arm will shift due to pressure differences.
Differential pressure manometers measure the height difference between the liquid levels, which corresponds to the pressure difference between the sources.
Gauge pressure manometers reference the atmospheric pressure in one arm of the manometer and display the height difference from that point, providing a gauge pressure reading.
Absolute pressure manometers incorporate a vacuum reference point, allowing for the measurement of absolute pressure.
By understanding these measurement types and how manometers exploit them, you gain a deeper appreciation for their ability to decipher the secrets of pressure.
Fluid Selection: The Right Liquid for Precise Manometer Readings
When it comes to choosing the fluid for a manometer, the selection depends on the specific application and the desired accuracy. Let’s delve into the key characteristics of different fluids and their suitability for various pressure measurement tasks.
Mercury: The Classic Choice
- High density: Mercury’s high density enables sensitive and precise pressure readings.
- Low vapor pressure: It minimizes fluid evaporation, ensuring stable readings.
- Toxicity: Notably, mercury is toxic, requiring careful handling and disposal.
Water: A Versatile Alternative
- Low density: Water provides less sensitivity than mercury but is suitable for measuring low pressures.
- Non-toxic: Water is safe for general use, making it ideal for educational and industrial settings.
- Fluctuating density: Temperature changes can impact water’s density, affecting accuracy.
Other Liquids: Tailored Solutions
Apart from mercury and water, various other liquids can be used in manometers for specialized applications.
- Alcohol: Less dense than water, alcohol offers increased sensitivity for measuring very low pressures.
- Carbon tetrachloride: Non-flammable and chemically inert, suitable for industrial settings with flammable fluids.
- Silicone oil: Resistant to high temperatures and chemical corrosion, ideal for use in harsh environments.
Choosing the Right Fluid
The choice of fluid for a manometer depends on the following factors:
- Accuracy requirements: High-density fluids like mercury provide greater accuracy.
- Pressure range: Fluctuations in density should be considered for fluids like water over a wide pressure range.
- Chemical compatibility: The fluid must be compatible with the gas being measured.
- Temperature stability: Density changes due to temperature variations should be accounted for.
By carefully selecting the appropriate fluid, you can optimize the performance of your manometer and obtain accurate and reliable pressure measurements.
Calibration and Accuracy: Ensuring Reliable Pressure Measurements
To ensure accurate pressure readings, proper calibration of manometers is crucial. Calibration involves comparing the readings of the manometer with a known reference pressure. This process verifies the precision and accuracy of the instrument throughout its measurement range.
During calibration, the manometer is subjected to a series of known pressures, and the observed readings are recorded. Any deviation from the reference pressure is then corrected by adjusting the scale or other components of the manometer.
Several factors can influence the accuracy of calibration:
- Fluid density: The density of the manometer fluid affects the pressure reading. Calibration should be performed using the exact fluid that will be used in the application.
- Temperature: Temperature variations can alter the density of the fluid, leading to inaccurate readings. Calibration should be carried out at the operating temperature or adjusted for temperature variations.
- Reference level: The reference level of the manometer must be precisely established to ensure accurate pressure measurements. Establishing a stable and accurate reference level is critical.
Regular calibration is essential to maintain the accuracy and reliability of manometers. By ensuring proper calibration, you can guarantee that your pressure measurements are precise and trustworthy.
Sensitivity and Inclined Manometers (Heading)
- Describe how inclined manometers provide greater sensitivity than vertical manometers.
- Explain the relationship between the slope of the inclined tube and the sensitivity of the manometer.
Sensitivity and Inclined Manometers
Manometers are pressure-measuring devices that utilize the principle of fluid statics. Vertical manometers, the most common type, consist of a vertical tube filled with a fluid. When pressure is applied to the tube, the fluid level rises or falls. The height difference, measured in units of length, indicates the pressure.
Inclined Manometers
Inclined manometers offer greater sensitivity than vertical manometers. Instead of a vertical tube, they have an inclined tube. This design amplifies the height difference for a given pressure change, allowing for more precise measurements.
Relationship between Slope and Sensitivity
The sensitivity of an inclined manometer is directly related to the slope of the inclined tube. A steeper slope results in a greater height difference for a given pressure change. This enhanced sensitivity makes inclined manometers ideal for applications requiring very accurate pressure measurements.
Advantages of Inclined Manometers
Inclined manometers offer several advantages over vertical manometers:
- Increased sensitivity: Amplifies the height difference, facilitating more precise measurements.
- Wide pressure range: Can measure both low and high pressures.
- Versatile: Suitable for various fluids, including liquids and gases.
Applications
Inclined manometers are commonly used in:
- Measuring small pressure differences
- Calibrating other pressure instruments
- Monitoring pressure in sensitive systems, such as medical devices and vacuum chambers
Choosing the Right Manometer
When selecting a manometer, consider the sensitivity and accuracy required for your application. If high precision is critical, an inclined manometer with a steep slope is recommended. For general-purpose use, a vertical manometer may suffice.
Reference Level: The Bedrock of Precise Pressure Readings
When measuring pressure using a manometer, establishing a reference level is paramount for accurate readings. It’s like having a fixed point from which all measurements begin, ensuring consistency and reliability.
The reference level is typically a horizontal plane set at a specific height in the manometer. This level serves as a baseline against which the height difference between liquid levels is measured. By determining the vertical distance between the reference level and the liquid levels, we can accurately calculate pressure.
Establishing the reference level requires careful attention. It’s done by aligning the zero point of the manometer scale with the liquid level in one of the manometer’s arms. This arm is typically open to the atmosphere, creating a reference pressure of zero.
Maintaining the reference level is also crucial. Any changes in the level will alter the pressure measurements. Factors like temperature fluctuations and fluid evaporation can affect the reference level, so regular checks and adjustments may be necessary.
By understanding and maintaining the reference level, you lay the foundation for accurate pressure measurements. It’s the cornerstone that ensures the reliability of your manometer readings.
Pressure Conversion (Heading)
- Discuss the different units of pressure and the conversion equations required to interpret pressure readings.
- Provide examples of conversions between common pressure units.
Pressure Conversion: Unraveling the Enigma of Pressure Units
Understanding pressure readings requires familiarity with the diverse units of pressure used across various industries. Pressure conversion is essential to interpret these readings and ensure accuracy in applications ranging from fluid dynamics to weather forecasting.
The most common pressure unit is the pascal (Pa), defined as the force of one newton applied perpendicularly to a surface of one square meter. However, other units like atmospheres (atm), pounds per square inch (psi), and millimeters of mercury (mmHg) are still widely used.
Converting pressure units requires the following equations:
- 1 atm = 101325 Pa
- 1 psi = 6894.757 Pa
- 1 mmHg = 133.322 Pa
For example, to convert 20 psi to pascals, we use the equation:
20 psi × 6894.757 Pa/psi = 137895.14 Pa
Common pressure unit conversions include:
- 1 atm = 14.696 psi
- 1 atm = 760 mmHg
- 1 psi = 51.715 mmHg
By understanding these conversion equations, engineers, scientists, and technicians can seamlessly interpret pressure readings from various instruments and sources.
Temperature’s Impact on Manometer Readings and Mitigation
In the realm of pressure measurement, temperature plays a crucial role that cannot be overlooked. Temperature directly affects the density of fluids, which, in turn, influences the pressure readings obtained from manometers.
As temperature increases, the density of most fluids decreases. This inverse relationship means that the same volume of fluid will weigh less at higher temperatures. In a manometer, this reduced density* translates into **lower pressure readings.
To compensate for temperature effects, several methods can be employed:
-
Choosing the Right Fluid: Some fluids exhibit minimal density variations with temperature changes. Selecting such fluids for manometers can minimize temperature-induced errors.
-
Temperature Correction: By knowing the fluid’s density-temperature relationship, corrections can be applied to pressure readings to account for temperature variations. This is especially important for precision measurements.
-
Using Compensated Manometers: Specialized manometers are designed with built-in temperature compensation mechanisms. These devices automatically adjust their readings based on temperature changes, providing accurate measurements across a wide temperature range.
By understanding and addressing temperature effects, you can ensure the reliability and accuracy of pressure measurements obtained from manometers. This knowledge empowers you to make informed decisions in various applications where precise pressure monitoring is critical.
Interpreting Liquid Level Readings (Heading)
- Describe the process of measuring the height difference between liquid levels in a manometer.
- Explain how to convert the height difference to pressure using fluid density.
Interpreting Liquid Level Readings in Manometers
When measuring pressure with a manometer, the key step lies in accurately interpreting the liquid level readings. This involves measuring the height difference between the liquid levels in the manometer’s tubes.
Determining the Height Difference
To measure the height difference, use a ruler or caliper to measure the vertical distance between the highest and lowest points of the liquid surfaces. Accuracy is crucial, so ensure a precise measurement.
Converting Height Difference to Pressure
Once the height difference is known, it can be converted to pressure by multiplying the height difference by the fluid density. Fluid density is a property of the liquid used in the manometer, typically mercury or water. The formula is:
Pressure = Height Difference × Fluid Density
Example
Consider a U-tube manometer containing mercury with a height difference of 10 cm. The density of mercury is approximately 13.6 g/cm³.
Pressure = 10 cm × 13.6 g/cm³
Pressure = 136 g/cm³
This result indicates that the pressure difference measured by the manometer is 136 g/cm³. By converting to other pressure units, such as pascal (Pa) or pounds per square inch (psi), the pressure value can be interpreted in different contexts.
Interpreting liquid level readings in manometers is a crucial step in accurately determining pressure. By measuring the height difference and utilizing the fluid density, users can convert these readings into meaningful pressure values. Careful attention to accuracy and understanding the principles involved ensure reliable pressure measurements in various applications.
Static and Dynamic Pressure (Heading)
- Define static and dynamic pressure and explain their relevance to manometer measurements.
- Discuss the limitations of manometers in measuring dynamic pressure.
Static and Dynamic Pressure: A Tale of Two Pressures
In the realm of manometers, we encounter two distinct types of pressure: static pressure and dynamic pressure. Static pressure measures the pressure of a fluid at rest, while dynamic pressure measures the pressure exerted by a moving fluid.
Manometers are primarily designed to measure static pressure. This type of pressure is uniform in all directions and unaffected by the fluid’s velocity. It is commonly used to measure the pressure in tanks, pipelines, and vessels.
Dynamic pressure, on the other hand, is generated by the fluid’s motion. It is dependent on the velocity of the fluid and is proportional to the square of the velocity. Manometers have limitations in measuring dynamic pressure because they are sensitive to changes in velocity, which can affect the accuracy of the readings.
This limitation arises from the design of manometers. The fluid in a manometer is at rest, creating a static pressure that is measured. When a moving fluid enters the manometer, it creates a dynamic pressure that cannot be accurately measured by the static pressure-measuring device.
To accurately measure dynamic pressure, specialized instruments such as Pitot tubes and impact probes are used. These instruments are designed to measure the velocity of the fluid and convert it to a pressure reading.
Safety Considerations (Heading)
- Outline potential safety hazards associated with manometers, such as spilled fluids and glass breakage.
- Describe recommended safety precautions to minimize risks.
Manometers: A Comprehensive Guide to Measuring Pressure
In the realm of fluid mechanics, manometers emerge as indispensable tools for measuring pressure. Their simplicity and versatility have made them a staple in various industries, from healthcare to manufacturing. Whether you’re a seasoned professional or an eager student, this comprehensive guide will provide you with an in-depth understanding of manometers and their applications.
Types of Manometers: Exploring the Options
Manometers come in different shapes and sizes, each tailored to specific measurement requirements. From vertical and inclined to U-tube and digital manometers, the choice depends on factors like accuracy, sensitivity, and the type of pressure being measured.
- Vertical Manometers: The classic vertical design stands tall, offering a straightforward method of pressure measurement. Its simple construction makes it ideal for quick and convenient readings.
- Inclined Manometers: Inclined manometers take precision to the next level. By tilting the measuring tube, they amplify small pressure differences, resulting in enhanced sensitivity.
- U-Tube Manometers: U-tube manometers, with their distinct U-shaped tube, provide exceptional accuracy for measuring differential pressure. The height difference between the liquid levels directly corresponds to the pressure difference.
- Digital Manometers: Digital technology brings modern convenience to pressure measurement. These devices employ electronic sensors to provide precise readings with ease of use and advanced features.
Measurement Types: Unlocking the Pressure Spectrum
Manometers aren’t restricted to a single type of pressure measurement. They can delve into the realms of differential, gauge, and absolute pressure:
- Differential Pressure: This measurement compares the pressure between two points, disregarding atmospheric pressure. Differential manometers, like U-tube manometers, excel in this domain.
- Gauge Pressure: Gauge pressure measures the pressure above atmospheric pressure. It’s widely used in industrial applications where atmospheric pressure serves as the reference point.
- Absolute Pressure: An absolute measurement takes into account both atmospheric pressure and the pressure being measured, providing a complete picture of pressure magnitude.
Fluid Selection: A Delicate Balancing Act
The choice of fluid used in a manometer is a critical factor influencing accuracy and suitability for specific applications. Mercury, with its high density, offers precise readings but raises safety concerns due to its toxicity. Water, on the other hand, is less precise but safer and more affordable. Other fluids, like oils and gases, may be used for specialized applications, such as measuring very low or high pressures.
Calibration and Accuracy: Ensuring Unwavering Precision
Calibration is the cornerstone of accurate manometer readings. Regular calibration against known pressure standards ensures that the manometer aligns perfectly with the true pressure values. Factors like temperature and fluid level can affect calibration; hence, careful attention should be given to maintaining optimal conditions.
Sensitivity and Inclined Manometers: Magnifying the Subtleties
Inclined manometers possess a unique advantage—their sensitivity. By adjusting the slope of the inclined tube, a smaller pressure difference results in a larger height difference in the liquid levels, enhancing the manometer’s ability to detect minute pressure changes.
Reference Level: The Foundation of Accurate Measurements
Establishing a precise reference level is crucial for reliable pressure readings. This reference point, often marked on the manometer, serves as the zero point for all pressure measurements, ensuring consistent and accurate results.
Pressure Conversion: A Universal Language
Pressure measurements can be expressed in various units, such as Pascals, pounds per square inch (psi), and atmospheres. Understanding the conversion equations is essential to interpret readings correctly and facilitate comparisons between different units.
Temperature Effects: Understanding the Fluid’s Response
Temperature can influence the density of the fluid used in the manometer, affecting the pressure readings. By understanding the fluid’s temperature dependence and applying appropriate corrections, accurate measurements can be maintained across varying temperatures.
Interpreting Liquid Level Readings: Deciphering the Height Difference
Measuring the height difference between the liquid levels in a manometer is the key to determining the pressure. Using the fluid’s density and the height difference, the pressure can be calculated with precision.
Static and Dynamic Pressure: Capturing the Fluctuations
Manometers primarily measure static pressure—the pressure in a fluid at rest. However, dynamic pressure, which occurs when a fluid is in motion, can also be measured using specialized techniques.
Safety Considerations: Prioritizing Well-being
Manometers, especially those containing mercury or glass components, require careful handling and safety precautions. Spilled fluids and glass breakage can pose hazards; hence, proper training, protective gear, and adequate ventilation should be ensured.
By embracing the guidance outlined in this comprehensive guide, you can confidently navigate the world of pressure measurement using manometers. From understanding different types and measuring techniques to ensuring accuracy and safety, this knowledge will empower you to harness the power of manometers for a wide range of applications.