Measuring Heat: Thermometers, Calorimetry, And Heat Transfer
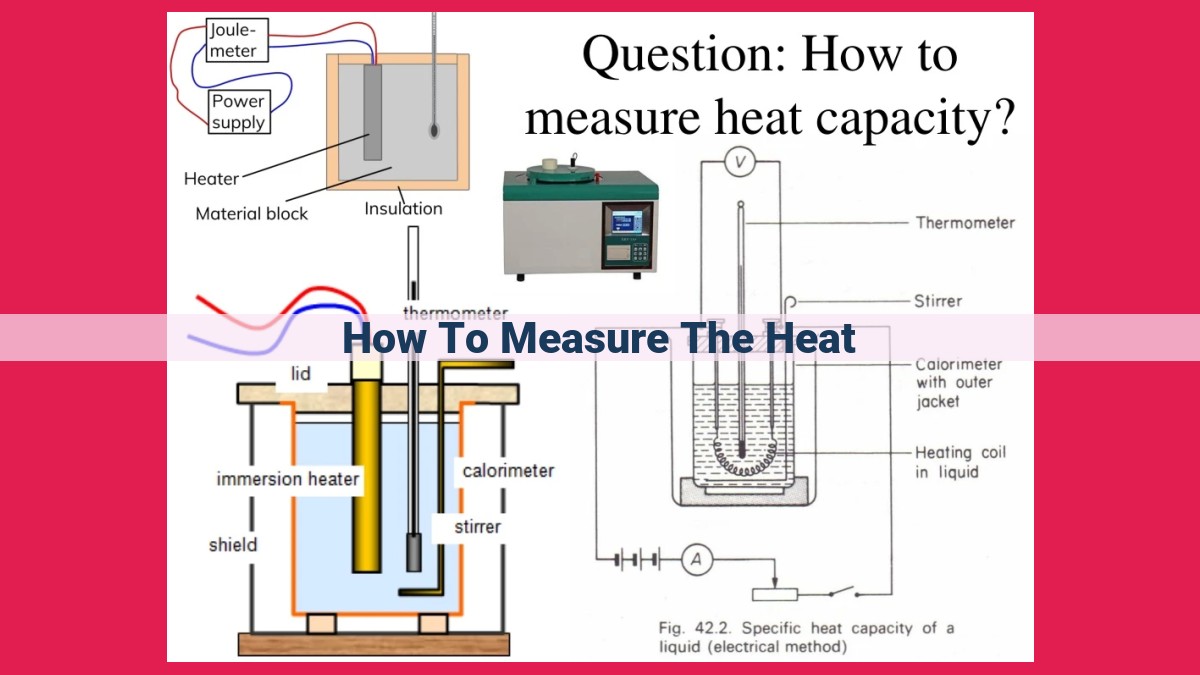
To measure heat, we first measure temperature using thermometers, which exploit thermal expansion. Heat is the transfer of thermal energy, and its measurement involves calorimetry. The rate of heat transfer depends on specific heat capacity, which describes the energy stored per unit mass of a substance for a given temperature change. Latent heat is the energy released or absorbed during phase changes, such as melting or freezing. Calorimetry allows us to accurately quantify heat transfer by measuring temperature changes and specific heat capacity.
Temperature: Measuring Hotness
In the realm of physics, temperature stands as a fundamental concept, quantifying the hotness or coldness of a substance. Understanding temperature is crucial for a myriad of scientific disciplines and everyday applications.
The Essence of Temperature
Temperature is a measure of the average kinetic energy of particles within a substance. As the particles move faster, the substance becomes hotter, indicating a higher temperature. Conversely, slower-moving particles result in a lower temperature.
Thermometer: The Standard of Measurement
The primary tool for measuring temperature is the thermometer. Thermometers utilize various physical properties that change with temperature, such as volume expansion, electrical resistance, and color change. By observing these changes, we can determine the temperature of the substance being measured.
Thermal Expansion: Heat’s Impact on Dimensions
Thermal expansion is a phenomenon where the physical dimensions of a substance increase with increasing temperature. This property finds practical applications in devices such as bimetallic strips used in thermostats and precision instruments like thermometers.
Heat Transfer: The Dance of Energy
Heat is the transfer of thermal energy between substances or objects. This energy flow occurs through three primary mechanisms: conduction, convection, and radiation. Conduction involves direct contact, convection relies on fluid movement, and radiation involves electromagnetic waves. Understanding heat transfer is essential for fields ranging from engineering to meteorology.
Heat: The Flow of Thermal Energy
In the realm of physics, heat stands as a pivotal force, the invisible hand that orchestrates the transfer of thermal energy between objects. It’s the driving force behind the warmth you feel from a crackling fire, the cooling sensation of a summer breeze, and the steady metamorphosis of ice into liquid water.
Measuring heat transfer is crucial to comprehending its transformative power. Enter the world of calorimeters, ingenious devices meticulously crafted to quantify this elusive energy exchange. By cleverly measuring the temperature changes within a meticulously calibrated system, calorimeters unveil the specific heat capacity of substances—a telltale property that governs how readily they absorb and release heat.
Specific heat capacity, measured in joules per gram per degree Celsius (J/g°C), acts as a fingerprint for each material, a unique identifier that reveals its ability to store thermal energy. Imagine a race, where different runners possess varying capacities to hold onto heat. Some, like metals, are sprinters, swiftly absorbing and dissipating heat. Others, like water, are marathoners, possessing an exceptional capacity to store vast amounts of thermal energy.
Understanding heat transfer and specific heat capacity is not merely an academic pursuit; it’s a key to unlocking a world of practical applications. From designing energy-efficient buildings to optimizing industrial processes, manipulating heat transfer empowers us to harness its power for our benefit.
Specific Heat Capacity: The Energy Storage Capacity
- Introduce the concept of specific heat capacity and its importance in understanding heat transfer.
- Describe methods for measuring specific heat capacity.
- Emphasize the role of specific heat capacity in thermal energy storage applications.
Specific Heat Capacity: The Energy Storage Champion
In the realm of thermal energy, where heat flows and temperatures rise, there exists a captivating property known as specific heat capacity. It’s a measure of how effectively substances absorb or release heat without causing a significant change in temperature. Simply put, it’s the energy required to raise the temperature of a unit mass of a substance by one degree Celsius or Kelvin.
Measuring specific heat capacity is a delicate yet crucial task. Ingenious methods have been devised, including the method of mixtures, where two substances of known heat capacities are combined and their final temperature is measured. This allows scientists to calculate the unknown specific heat capacity.
The significance of specific heat capacity lies in its profound influence on thermal energy storage applications. Substances with high specific heat capacities act like thermal sponges, absorbing large amounts of heat before their temperature noticeably increases. This makes them indispensable in applications such as thermal batteries, heat sinks, and phase-change materials used in energy-efficient buildings.
Conversely, substances with low specific heat capacities are less effective at storing thermal energy. They release or absorb heat more readily, making them suitable for applications where rapid temperature changes are desired, such as heat exchange fluids or cooling systems.
Understanding specific heat capacity is like unlocking the secrets of energy storage. It empowers us to tailor materials and design systems that optimize thermal efficiency, reduce energy consumption, and pave the way for innovative thermal technologies in the years to come.
Latent Heat: Unveiling the Hidden Energy of Phase Changes
In the realm of thermodynamics, where energy flows and transformations occur, a fascinating phenomenon known as latent heat plays a pivotal role. It is the epitome of energy in disguise, concealed within the depths of matter during phase transitions.
Defining Latent Heat: The Hidden Energy
Latent heat is the energy absorbed or released by a substance during a phase change while its temperature remains constant. It is aptly named “latent,” meaning “hidden,” as it is not readily apparent in the form of increased temperature. Phase changes include the transformation of solids to liquids (melting), liquids to gases (vaporization), and vice versa.
Linking Specific Heat and Latent Heat: A Dynamic Duo
Latent heat is inherently linked to another thermodynamic property called specific heat capacity. Specific heat capacity measures the amount of heat energy required to raise the temperature of a substance by one degree Celsius. During a phase change, once the substance reaches its melting or boiling point, it absorbs heat not to elevate its temperature further but to facilitate the phase transition. This absorbed energy manifests as latent heat.
The Significance of Latent Heat: A Thermal Powerhouse
Latent heat has profound implications in numerous applications. One notable example is in the field of thermal energy storage. Materials with high latent heat capacities, known as phase-change materials (PCMs), absorb and release large amounts of energy during phase transitions. This makes them ideal for storing thermal energy for applications such as thermal energy management in buildings and the development of compact and efficient thermal storage systems.
In summary, latent heat is a fundamental concept in thermodynamics, providing an insight into the energy dynamics of phase changes. Its relationship with specific heat capacity unveils the hidden energy exchange during these transitions. Understanding latent heat is crucial for diverse applications, particularly in thermal energy storage, where it plays a pivotal role in harnessing and utilizing heat energy in innovative ways.
Calorimetry: Quantifying Heat Transfer
- Describe the principles of calorimetry and its applications in measuring heat transfer.
- Explain the use of calorimeters to determine specific heat capacity and latent heat.
- Emphasize the importance of calorimetry in understanding thermal energy exchange.
Calorimetry: Quantifying the Invisible
In the realm of energy, understanding how heat flows is crucial. Calorimetry is the art of measuring this enigmatic heat transfer. It provides scientists and engineers with a powerful tool to quantify the energy hidden within materials and the exchanges that occur when they interact.
At its core, calorimetry is founded on the principle of energy conservation. Heat, an invisible flow of thermal energy, can neither be created nor destroyed. Instead, it can be transferred from one object to another, changing their temperatures. Calorimeters, specialized devices, harness this principle to capture and measure the heat exchanged.
One common type of calorimeter is the bomb calorimeter. It’s a sealed chamber where a substance is burned in the presence of water. The temperature rise of the water, measured precisely, reveals the heat released by the burning substance. This information is essential for determining the specific heat capacity of materials, a measure of their ability to store thermal energy.
Another type of calorimeter is the differential scanning calorimeter (DSC). This device measures the heat flow into or out of a sample as it undergoes changes in temperature. DSC is used to study phase transitions, such as melting and freezing, where heat is released or absorbed without a change in temperature. By analyzing these heat flow patterns, scientists can determine the latent heat associated with phase changes, a key factor in thermal energy storage applications.
Calorimetry is a powerful tool that enables us to quantify the hidden energy in materials and processes. It deepens our understanding of thermal energy exchange, paving the way for advancements in heating and cooling technologies, energy efficiency, and our overall comprehension of the world around us.