Maximizing Cell Growth: Optimizing Surface Area-To-Volume Ratio For Efficient Nutrient Transport
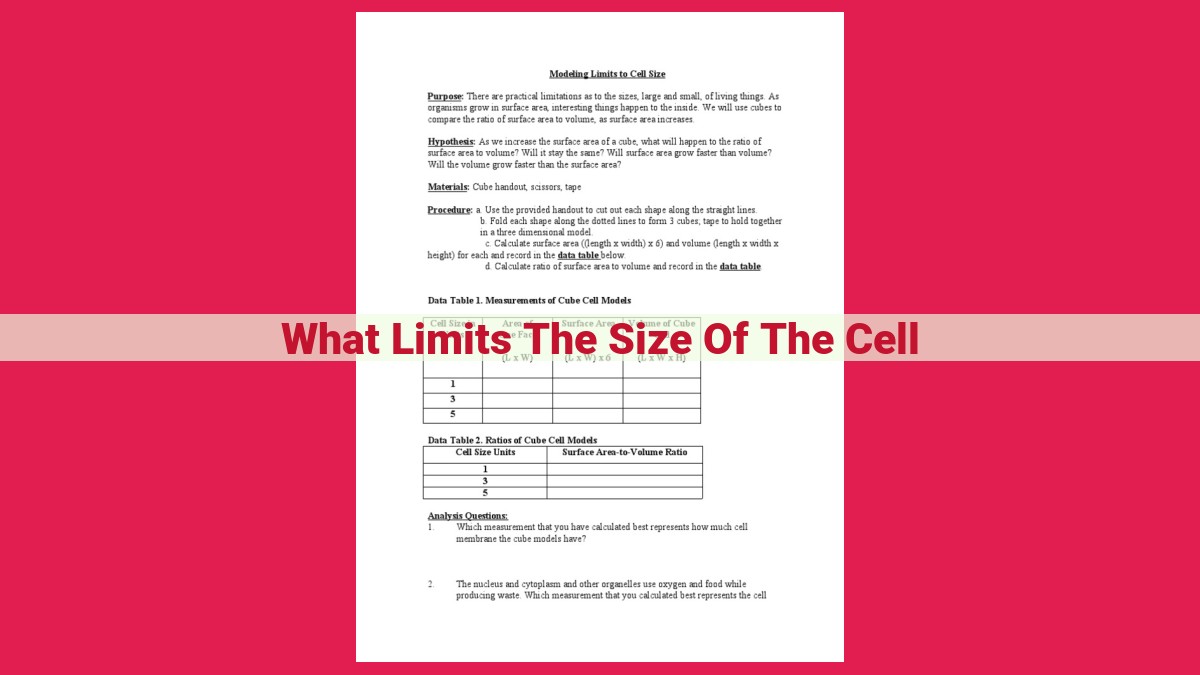
Cell size is constrained by the surface area-to-volume ratio, which impacts diffusion rates and nutrient availability. As cells increase in size, their surface area-to-volume ratio decreases, leading to slower diffusion and potential nutrient starvation. Additionally, increasing cell size elevates cytoplasmic viscosity, affecting organelle efficiency and structural stability. These factors collectively limit cell growth to ensure efficient nutrient acquisition, metabolic function, and structural integrity.
Surface Area-to-Volume Ratio: The Crux of Cell Size
In the microscopic world of cells, size plays a pivotal role in their ability to thrive. One crucial factor that governs cell size is the surface area-to-volume ratio. This ratio is a determinant of the cell’s ability to exchange nutrients and waste products with its surroundings, a process essential for its survival and growth.
As a cell grows in volume, its surface area increases at a slower rate. This inverse relationship between surface area and volume creates a challenge: the larger the cell, the smaller its surface area-to-volume ratio. This ratio has significant implications for the cell’s ability to meet its metabolic needs.
Diffusion Rate: Essential for Nutrient Acquisition
Diffusion, the passive movement of molecules from areas of higher to lower concentration, is crucial for nutrient acquisition by cells. The surface area-to-volume ratio plays a critical role in determining diffusion efficiency. A large surface area relative to its volume allows the cell to take up more nutrients from its surroundings. Conversely, a small surface area-to-volume ratio limits nutrient uptake.
Nutrient Availability: Fueling Cell Growth
The availability of nutrients is directly tied to the surface area-to-volume ratio and diffusion rate. Cells with a large surface area-to-volume ratio have a greater capacity to take up nutrients, supporting their growth and function. However, as a cell grows, its surface area-to-volume ratio decreases, potentially limiting nutrient supply. This limitation can hinder cell growth and impair cellular processes.
Diffusion Rate: The Lifeline for Nutrient Acquisition
Imagine a bustling city, where people and goods are constantly moving. If the roads are wide and spacious, transportation is efficient, allowing for a smooth flow of resources. However, as the city grows larger, the roads remain the same, resulting in traffic congestion and delayed deliveries. This is similar to what happens within cells as they increase in size.
In cells, the surface area-to-volume ratio is crucial for diffusion, the movement of molecules across a concentration gradient. As cells grow, their volume increases more rapidly than their surface area, leading to a decreased surface area-to-volume ratio. This inverse relationship has a significant impact on diffusion efficiency.
Imagine a cell like a sponge with a large surface area. The more surface area the sponge has, the easier it is for water to diffuse into its pores. However, as the sponge grows larger, its surface area relative to its volume decreases, making it harder for water to penetrate its interior.
This decrease in surface area-to-volume ratio affects the diffusion rate, or the speed at which molecules move across the cell membrane. A slow diffusion rate hinders the delivery of nutrients to the cell’s interior and the removal of waste products. This can lead to nutrient starvation and waste accumulation, impairing cell growth and function.
Therefore, the surface area-to-volume ratio plays a vital role in maintaining a sufficient diffusion rate for nutrient acquisition. As cells grow, they must either increase their surface area or decrease their volume to maintain an efficient diffusion rate and ensure a steady supply of nutrients for proper function.
Nutrient Availability: Fueling Cell Growth
In the bustling metropolis of the cell, nutrient availability plays a pivotal role in fueling growth and sustaining life. Just like a city’s infrastructure must efficiently deliver resources to its inhabitants, the cell’s surface area-to-volume ratio and diffusion rate act as vital pipelines, ensuring a steady supply of nutrients for its internal machinery.
As a cell grows in size, its surface area—the gateway for nutrient exchange—increases at a slower pace than its volume, where nutrients are consumed. This disparity leads to a decreasing surface area-to-volume ratio, creating a logistical challenge for nutrient acquisition. Nutrients, like miniature delivery trucks, must navigate through the cell’s enlarged interior to reach their designated destinations. As a result, diffusion, the process by which nutrients move from areas of high concentration to low concentration, becomes less efficient.
The sluggish diffusion rate further exacerbates the nutrient deficit. Nutrients, akin to essential fuel, are unable to reach their target organelles in sufficient quantities to support cell growth and function. This nutrient starvation can lead to a ripple effect, impairing metabolic processes, hindering organelle efficiency, and ultimately compromising cell health.
Therefore, maintaining an adequate surface area-to-volume ratio and diffusion rate is paramount for nutrient availability. These key factors ensure that nutrients, the lifeblood of the cell, can be efficiently delivered to fuel cell growth and power its vital functions.
Organelle Efficiency: The Vital Cogs of the Cell Machinery
At the very heart of every cell lies a complex network of organelles, each tirelessly performing specialized tasks to sustain cellular life. However, the efficiency of these organelles is directly intertwined with the overall cell size and metabolic activity.
As cell size increases, cytoplasmic viscosity thickens, creating a viscous environment that hinders organelle movement. This can significantly impair the efficiency of nutrient processing, as organelles find it increasingly difficult to navigate the cytoplasm to access essential molecules. Additionally, metabolic rate, which increases with cell size, further exacerbates organelle inefficiency. The heightened cellular activity generates more byproducts and waste, further taxing the limited mobility of organelles.
The Consequences of Impaired Organelle Efficiency
Stalled organelles have far-reaching consequences for cellular function. Impaired nutrient processing can lead to nutrient starvation, hindering cell growth and division. Accumulated waste products can create a toxic environment within the cell, disrupting normal cellular processes and potentially triggering apoptosis (programmed cell death).
Moreover, decreased organelle mobility affects not only nutrient uptake but also the distribution of cellular components. This can disrupt the delicate balance of molecular interactions and signaling pathways within the cell, compromising cellular stability and compromising its ability to respond to external cues.
To maintain optimal organelle efficiency, cells have evolved various strategies. One such strategy is to increase the surface area-to-volume ratio by increasing the number of cell membrane folds or creating extensive networks of internal membranes. This maximizes the contact area between the cell and its surroundings, facilitating nutrient uptake and waste removal.
Another strategy involves regulating cytoplasmic viscosity. Cells can adjust the concentration of macromolecules and cytoskeletal elements to fine-tune the viscosity of the cytoplasm. This allows for optimal organelle mobility while maintaining cellular structural stability.
Maintaining Cellular Harmony Through Organelle Efficiency
Organelle efficiency is a critical aspect of cellular health and function. It is intricately linked to cell size, metabolic rate, and cytoplasmic viscosity to ensure a delicate balance that supports cellular growth, division, and survival. By understanding the intricacies of organelle efficiency, we gain a deeper appreciation for the remarkable complexity and resilience of living cells.
Metabolic Rate: Balancing Growth and Stability
In the intricate realm of cell biology, metabolic rate plays a crucial role in maintaining the delicate equilibrium between growth and stability. This intricate balance is orchestrated by a complex interplay of surface area-to-volume ratio, diffusion rate, and nutrient availability.
Surface Area to Volume Ratio: The Foundation of Metabolic Demand
The surface area-to-volume ratio of a cell determines its capacity to exchange nutrients and waste products with its surroundings. As cells grow larger, this ratio decreases, reducing the efficiency of diffusion and nutrient acquisition. Consequently, larger cells face a greater metabolic demand to maintain their cellular functions.
Diffusion Rate: The Lifeline of Nutrient Supply
Diffusion rate, governed by the surface area-to-volume ratio, is essential for transporting nutrients across the cell membrane and eliminating waste. Slower diffusion rates hamper nutrient intake and waste removal, leading to nutrient starvation and toxic waste accumulation.
Nutrient Availability: Fueling Cell Growth and Function
The availability of nutrients is directly influenced by surface area-to-volume ratio and diffusion rate. Nutrient limitation can hinder cell growth and impair cellular processes, as essential resources become scarce. Maintaining an adequate supply of nutrients is paramount for optimal cell function and tissue health.
Cytoplasmic Viscosity: The Thickening Barrier
- Explain how cytoplasmic viscosity influences organelle efficiency and structural stability.
- Describe the effects of increased viscosity on organelle movement, nutrient distribution, and cell integrity.
Cytoplasmic Viscosity: The Thickening Barrier
Imagine yourself as a microscopic adventurer, exploring the bustling metropolis of a cell. Amidst the swirling organelles and nutrient-rich environment, you encounter a peculiar phenomenon: cytoplasmic viscosity. It’s like navigating through a thick, honey-like substance, hindering your every move.
Organelle Efficiency and Structural Stability
This viscosity plays a crucial role in the cell’s inner workings. As the cell grows and its metabolic rate increases, the cytoplasm thickens, slowing down the movements of organelles. Picture tiny motor-driven vehicles struggling to navigate through a viscous traffic jam. This reduced mobility impairs the efficiency of organelles responsible for nutrient processing, waste removal, and other vital cellular functions.
Moreover, increased viscosity can also affect the cell’s structural integrity. As organelles become sluggish, they struggle to maintain their positions within the cytoplasm. The cell’s internal framework weakens, increasing the risk of collapse and loss of function. It’s like a building with a weakened foundation, teetering on the brink of structural failure.
Nutrient Distribution and Organelle Immobility
The thickening cytoplasm also disrupts the distribution of nutrients and waste products within the cell. As organelles become bogged down in the viscous environment, they have difficulty transporting nutrients to where they’re needed and removing waste products. Imagine a mail carrier trying to deliver packages through a dense fog, his progress hindered at every turn. This impaired nutrient distribution can lead to starvation and accumulation of waste, creating a toxic environment for the cell.
As the viscosity increases, organelles become trapped, further impeding their ability to perform their tasks. It’s like a group of workers stuck in a crowded elevator, unable to reach their workstations. This immobility can lead to a breakdown of cellular processes and a decline in overall cell health.
Consequences for the Cell
The implications of increased cytoplasmic viscosity are far-reaching. It can slow down cell growth, reduce metabolic efficiency, and compromise structural integrity. In extreme cases, it can lead to cell death. Therefore, it’s essential for cells to tightly regulate their viscosity to ensure optimal function and maintain cellular homeostasis.
Structural Stability: Preserving Cell Integrity
Cell integrity is paramount for the proper functioning and survival of any cell. It ensures that the cell maintains its shape, protects its internal contents, and facilitates essential cellular processes. Structural stability is intricately linked to metabolic rate and cytoplasmic viscosity.
In cells with high metabolic rates, the increased production of energy and waste products can lead to a thickening of the cytoplasm. This increased viscosity can hinder the movement of organelles, which are essential for carrying out cellular functions. The reduced organelle mobility can impair nutrient processing and waste removal, further compromising cell integrity.
Moreover, excessive cytoplasmic viscosity can exert mechanical stress on the cell membrane and other cellular structures. This stress can weaken the cell’s ability to withstand external forces, making it more prone to rupture or collapse. Consequently, maintaining a balance between metabolic rate and cytoplasmic viscosity is crucial for preserving cell integrity.
The structural stability of a cell is also influenced by the cytoskeleton. The cytoskeleton is a network of protein filaments that provides mechanical support and shape to the cell. A well-organized cytoskeleton helps to maintain cell shape, resists mechanical stress, and facilitates cell movement. Disruptions in the cytoskeleton, such as those caused by certain drugs or diseases, can lead to loss of cell integrity and impaired cell function.
Therefore, maintaining cell integrity is essential for the proper functioning and survival of cells. By understanding the interplay between metabolic rate, cytoplasmic viscosity, and the cytoskeleton, researchers can gain valuable insights into the mechanisms that govern cell size, shape, and stability.