Mastering Rate-Determining Step Identification For Faster Reaction Understanding
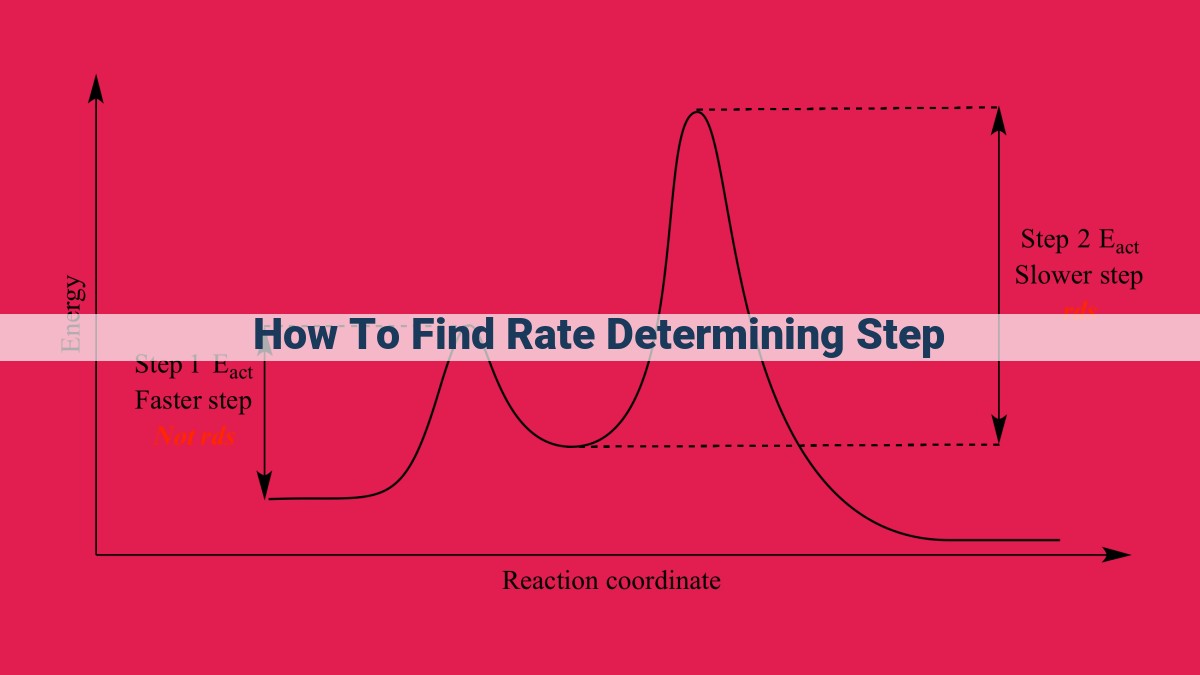
To find the rate-determining step (RDS), analyze the reaction mechanism and identify the slowest step, which governs the overall reaction rate. Examine the rate law to determine the concentration dependence of each step. Elementary steps and assumptions like steady state and pre-equilibrium can help identify the RDS. The rate law, activation energy, and transition state theory provide insights into the RDS’s order, energy barrier, and mechanism. Principles of microscopic reversibility and detailed balance further assist in finding the RDS in complex reactions.
What is the Rate-Determining Step (RDS)?
- Define the RDS as the slowest step in a reaction that determines the overall rate.
- Explain its significance in understanding reaction mechanisms.
Chapter 1: The Rate-Determining Step: A Crucial Gateway to Understanding Reaction Mechanisms
In the captivating world of chemistry, reactions are like intricate dance sequences, with molecules gracefully colliding and transforming into new creations. But within these mesmerizing moves, there’s a crucial step that dictates the overall tempo of the dance: the Rate-Determining Step (RDS).
The RDS is the slowest step in a reaction mechanism, the rate-limiting factor that sets the pace for the entire dance. Identifying the RDS is like finding the pivot point of a lever; it unlocks a deeper understanding of the reaction’s mechanism and allows us to predict and control its outcome.
Significance of the RDS
The RDS plays a pivotal role in unraveling the mysteries of reaction mechanisms. By pinpointing the RDS, we can:
- Comprehend how molecules interact and transform during a reaction.
- Determine the factors that influence the reaction rate, such as temperature and concentration.
- Predict the types of products formed and their relative proportions.
- Design and optimize chemical processes for specific applications.
Identifying the Rate-Determining Step Using Concentration Dependence: Unveiling the Bottleneck in Chemical Reactions
In the realm of chemistry, understanding why reactions proceed at different rates is crucial. One key factor that governs the overall pace of a reaction is the rate-determining step (RDS). Identifying the RDS is like finding the bottleneck in a manufacturing process, as it dictates the speed at which products are formed.
One powerful tool for identifying the RDS is by examining the rate law of the reaction. The rate law expresses the relationship between the reaction rate and the concentrations of the reactants. By studying the rate law, we can determine which step in the reaction mechanism is most sensitive to changes in reactant concentrations.
For example, consider the following reaction mechanism:
A + B -> C -> D + E
The first step (A + B -> C) is slow and the second step (C -> D + E) is fast. If we measure the reaction rate at varying concentrations of A and B, we will find that the rate law is first order in both A and B. This indicates that the first step is the rate-determining step, as it is the slowest step and the one that most strongly influences the overall reaction rate.
Another example is the SN2 reaction, where a nucleophile attacks a substrate to replace a leaving group. The rate law for an SN2 reaction is second order, meaning that the rate is proportional to the concentrations of both the nucleophile and the substrate. This suggests that the RDS involves the collision between the nucleophile and the substrate, which is the slowest step in the mechanism.
By carefully analyzing the rate law and understanding the reaction mechanism, we can pinpoint the RDS. This knowledge allows us to optimize reaction conditions, predict reaction rates, and design efficient synthetic pathways.
Elementary Steps and the Rate-Determining Step (RDS)
In any chemical reaction, the overall reaction rate is dictated by its slowest step, known as the rate-determining step (RDS). This key step governs how fast the reaction proceeds. To understand the RDS, it’s essential to break down the reaction into its individual steps, called elementary steps.
Each elementary step represents a specific reaction between specific reactants. The RDS is the slowest elementary step in the overall reaction mechanism. Identifying the RDS is crucial because it provides insights into the reaction’s rate and its dependence on different factors.
How to Identify the RDS
To determine the RDS, we examine the elementary steps involved in the reaction mechanism. The step that has the highest activation energy is typically the RDS. Activation energy represents the minimum energy required for a reaction to occur. A higher activation energy means that the reactants have to overcome a greater energy barrier to reach the transition state, making the reaction slower.
Once the RDS is identified, we can better understand the overall reaction rate and how it is affected by various factors such as temperature, concentration, and catalysts.
The Steady State Approximation and the Rate-Determining Step
In the realm of chemical reactions, the rate-determining step (RDS) reigns supreme as the slowest dance partner in the intricate choreography of a reaction mechanism. Identifying this crucial step is paramount in comprehending the symphony of molecular transformations. Among the tools at our disposal is the steady state approximation, a clever strategy that unlocks secrets from the chaos of reactions.
The steady state approximation is like a spotlight that illuminates the fleeting dance moves of intermediate species, which are the ephemeral molecules that fleetingly grace the reaction stage. This approximation assumes that, at some point during the dance, the concentration of these elusive intermediates remains relatively constant. It’s as if they’re stuck in a perpetual loop, constantly being created and consumed at the same rate.
This assumption might seem like a leap of faith, but it often reflects the reality of many reactions. Think of a relay race, where runners hand off the baton in a continuous flow. The number of runners on the track may fluctuate, but over time, the overall rate of the race remains steady. Similarly, in a reaction mechanism, the concentration of intermediates might fluctuate, but under steady state conditions, their average concentration remains constant.
The steady state approximation simplifies the complex dance of reactions by allowing us to focus on the steps that are truly impactful. By assuming that the concentration of intermediates is constant, we can eliminate them from the rate law equation. This simplification makes it easier to identify the RDS, as the rate law will only reflect the concentration dependence of the reactants and products.
Imagine a reaction mechanism with a series of elementary steps, each with its own unique rate constant. The RDS is the step with the highest activation energy, which means it’s the slowest and most difficult for the reactants to overcome. Under steady state conditions, the rate of the RDS becomes the rate-limiting factor for the entire reaction, and the rate law will directly reflect the rate of the RDS.
By applying the steady state approximation, we can simplify complex reaction mechanisms, isolate the RDS, and gain insights into the molecular choreography that governs chemical transformations. It’s like having a backstage pass to the intricate dance of reactions, allowing us to witness the hidden steps that shape the overall outcome.
Pre-Equilibrium Approximation and the Rate-Determining Step
In unraveling the mysteries of complex reaction mechanisms, chemists often encounter situations where reactants reach a state of equilibrium before the actual chemical transformation takes place. This delicate balance can obscure the true rate-determining step (RDS), the slowest step that governs the overall reaction rate.
The pre-equilibrium approximation offers a clever solution to this dilemma. It assumes that the reactants are in equilibrium before the RDS occurs, allowing us to simplify the reaction mechanism and identify the RDS more easily. This approximation is particularly useful when the equilibrium constant for the pre-equilibrium step is very large, indicating that the reactants are almost entirely converted to products.
Let’s take an example to illustrate how the pre-equilibrium approximation can help us pinpoint the RDS. Consider the following reaction:
A + B ⇌ C (fast equilibrium)
C → D (slow)
In this case, the first step establishes a rapid equilibrium between reactants A and B and intermediate C. The second step, the conversion of C to D, is the slow, rate-determining step.
Using the pre-equilibrium approximation, we can assume that the equilibrium between A and B is established before the RDS. This means that the concentration of C is essentially constant, as it is constantly being re-established by the fast equilibrium.
As a result, the rate law for the overall reaction is:
Rate = k[D]
where k is the rate constant for the RDS. This simplified rate law clearly shows that the RDS is the conversion of C to D, even though the actual reaction mechanism involves multiple steps.
The pre-equilibrium approximation is a valuable tool that helps chemists unravel the complexities of reaction mechanisms and identify the RDS, even in cases where reactants are in equilibrium. By assuming that the equilibrium is established before the RDS, we can simplify the mechanism and gain insights into the factors that control the overall reaction rate.
The Rate Law and the RDS
- Explain the concept of a rate law and its relationship to the RDS.
- Discuss how the rate law can provide insights into the RDS and its order of reaction.
The Rate Law and the Rate-Determining Step
In the realm of chemical reactions, the rate law plays a crucial role in deciphering the secrets of how fast a reaction proceeds. This enigmatic equation provides a mathematical blueprint that reveals the rate-determining step (RDS), the sluggish gatekeeper that governs the tempo of the entire reaction.
The rate law, a mathematical expression, depicts the relationship between the concentration of reactants and the rate of the reaction. Each reactant’s concentration is raised to a power, known as its order, reflecting its influence on the reaction rate. Intriguingly, the order of a reaction often mirrors the molecularity of its RDS, revealing the number of molecules directly involved in the rate-limiting step.
By delving into the rate law, chemists can uncover the mechanism of a reaction, the intricate dance of elementary steps that lead to the final products. The rate-determining step, with its languid pace, emerges as the key choreographer, dictating the overall rhythm of the reaction. Understanding the RDS is essential for gaining insights into the reaction’s dynamics and manipulating its rate.
Activation Energy and the Rate-Determining Step (RDS)
In the intricate world of chemical reactions, the Rate-Determining Step (RDS) plays a pivotal role in governing the overall reaction rate. It’s the slowest step in the reaction mechanism, like a gatekeeper that controls the pace at which molecules transform. Imagine a relay race, where runners represent individual reaction steps. The RDS is the slowest runner, setting the speed limit for the entire team.
The activation energy is an essential concept in understanding the RDS. It’s the energy barrier molecules must overcome to transform into products. Think of it as an obstacle course in a race. The higher the activation energy, the more challenging it is for molecules to clear the hurdle.
The activation energy of the RDS has a direct impact on the overall reaction rate. The higher the activation energy, the slower the reaction. This relationship is described by the Arrhenius equation, which shows that the reaction rate is exponentially dependent on the activation energy.
So, if you want to speed up a reaction, you can try reducing the activation energy of the RDS. One common strategy is using catalysts, which provide an alternative reaction pathway with a lower activation energy. It’s like giving the reaction a shortcut, allowing it to bypass the bottleneck created by the RDS.
Understanding the RDS is crucial for chemists seeking to control and optimize reactions. By manipulating activation energies, scientists can tailor reaction rates to suit various applications, from drug discovery to energy production.
Transition State Theory and the RDS
Imagine you’re driving a car up a steep hill. Getting to the top, the peak, represents the transition state of the reaction. Just like getting to the top of the hill is the slowest part of your journey, the rate-determining step (RDS) is the slowest step in a chemical reaction.
Transition state theory tells us that as reactants transform into products, they must pass through this transition state—a high-energy, unstable state resembling both reactants and products. The activation energy is the energy required to reach this transition state.
The RDS is the elementary step with the highest activation energy. This is the rate-limiting step that dictates the overall pace of the reaction. By studying the transition state, we gain insights into the nature of the RDS.
For example, if the transition state looks more like the reactants than the products, we deduce that the RDS involves breaking bonds in the reactants. Conversely, if the transition state resembles the products, the RDS likely involves forming bonds between products.
So, just like understanding the peak of the hill helps us navigate a winding road, transition state theory guides us in deciphering the intricate mechanisms and identifying the gatekeeper step—the RDS—that governs the pace of chemical reactions.
Microscopic Reversibility and the Rate-Determining Step (RDS)
The world of chemical reactions is a bustling metropolis, where molecules collide, transform, and create new substances. But within this chaotic dance, there’s an underlying order, a symphony of motion governed by the principle of microscopic reversibility.
Microscopic reversibility postulates that for every reaction that occurs in the forward direction, there’s an equal and opposite reaction that can proceed in the reverse direction, under the same conditions. This principle has profound implications for understanding the rate-determining step (RDS) of a chemical reaction.
Imagine a reaction mechanism as a winding road, where each step represents a different turn. The RDS is the slowest of these turns, the bottleneck that ultimately limits the overall rate of the reaction. Now, if we could run the reaction in reverse, the RDS would become the fastest turn, allowing the reverse reaction to proceed smoothly.
This interplay between forward and reverse reactions provides a powerful tool for identifying the RDS. By examining the reversibility of individual reaction steps, we can narrow down which step is likely to be the slowest and thus the RDS.
For example, consider the following reaction mechanism:
A + B → C + D
C + D → A + B
In this mechanism, both steps are reversible. However, if the second step is much slower than the first, then it is likely to be the RDS. This is because the reverse of the second step is the forward of the first step, and the principle of microscopic reversibility tells us that these two steps should have comparable rates.
Therefore, by analyzing the reversibility and relative rates of individual reaction steps, we can leverage microscopic reversibility to identify the RDS and gain a deeper understanding of the intricate mechanisms that govern chemical reactions.
Unlocking the Secrets of Chemical Reactions: Exploring the Rate-Determining Step
Every chemical reaction unfolds like a carefully choreographed dance, where molecules interact in a sequence of steps. Imagine the dance floor as a reaction mechanism, and each step as an elementary reaction. However, not all steps are created equal. There’s always one that steals the show – the rate-determining step (RDS) – the slowest step that sets the pace for the entire reaction.
Catch the Culprit: Identifying the RDS
Like detectives in a crime scene, chemists use various techniques to identify the RDS. One such method is examining concentration dependence. Each step in a reaction mechanism has its own concentration dependence, and the RDS is the step that shows the strongest dependence on the concentration of its reactants.
Another approach involves analyzing elementary steps. By breaking down the reaction mechanism into its individual steps, we can pinpoint the step with the highest activation energy. This step is often the RDS, as it’s the most challenging to overcome energetically.
Approximating the Truth: Steady State and Pre-equilibrium
Sometimes, reaction mechanisms are too complex to unravel all at once. That’s where approximations come in. The steady state approximation assumes that the concentration of certain intermediates remains constant during the reaction. This assumption simplifies the mechanism and allows us to identify the RDS more easily.
Similarly, the pre-equilibrium approximation assumes that certain steps are so fast that they reach equilibrium before the rest of the reaction. By making this assumption, we can simplify the mechanism and pinpoint the RDS.
The Rate Law and the RDS: A Tale of Two
The rate law of a reaction provides a mathematical expression that describes the relationship between the rate of the reaction and the concentrations of the reactants. By analyzing the rate law, we can determine the order of the reaction – the exponent to which the reactant concentrations are raised. The order of a reaction often corresponds to the molecularity of the RDS – the number of molecules involved in the RDS.
Microscopic Reversibility and Detailed Balance: Unmasking the Hidden Truth
In the world of chemistry, actions often have an equal and opposite reaction. This principle, known as microscopic reversibility, implies that for every step in a reaction mechanism, there is a reverse step. By analyzing the microscopic reversibility of the steps, we can sometimes identify the RDS.
Detailed balance takes microscopic reversibility a step further. It predicts that the rate of the forward reaction is equal to the rate of the reverse reaction at equilibrium. This principle can be used to derive rate laws and identify the RDS in complex reaction mechanisms.
By unraveling the secrets of the RDS, we gain a deeper understanding of the intricate dance of chemical reactions. This knowledge empowers us to predict reaction rates, design experiments, and even manipulate reaction pathways for desired outcomes. So, next time you witness a chemical reaction, take a moment to appreciate the rhythm and flow of the molecular dance, and remember the importance of the rate-determining step – the maestro that sets the pace for the entire symphony.