Mastering Enthalpy Change (Δh): A Comprehensive Guide
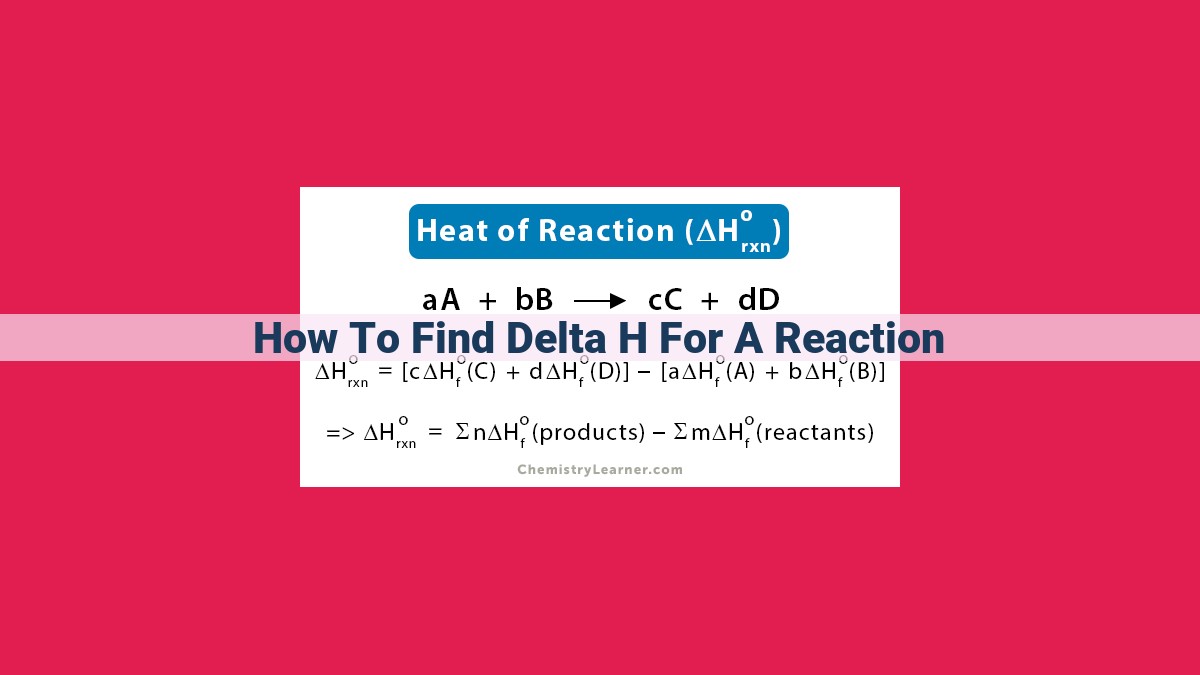
To find the enthalpy change (ΔH) for a reaction, understand enthalpy and measure it calorimetrically or via Hess’s Law. Determine the reaction type (exothermic or endothermic) based on the ΔH sign. Calculate ΔH using standard enthalpies of formation: ΔHr° (products) – ΔHr° (reactants) = ΔH. Applications of ΔH include predicting reaction spontaneity, optimizing chemical processes, and understanding energy changes.
Exploring the Enigmatic World of Enthalpy Change (ΔH)
Unlocking the secrets of chemical reactions lies in comprehending the captivating concept of enthalpy change. Enthalpy (H) encapsulates the total energy of a system, encompassing its thermal energy, as well as potential energy stored within chemical bonds. When chemical transformations occur, enthalpy change (ΔH) measures the variation in enthalpy, revealing the energy absorbed or released during the process.
Understanding ΔH is paramount in deciphering the significance of chemical reactions. A positive ΔH indicates an endothermic reaction, where the system absorbs energy from its surroundings to break bonds and create new ones. Conversely, an exothermic reaction is characterized by a negative ΔH, signifying the release of energy as bonds are formed and broken.
Two crucial concepts in the realm of enthalpy change are enthalpy of formation and standard enthalpy of formation. Enthalpy of formation represents the enthalpy change when one mole of a substance is formed from its constituent elements under standard conditions (298 K, 1 atm). Standard enthalpy of formation is the enthalpy change when one mole of a substance is formed from its elements in their standard states. These values, tabulated for a wide range of substances, serve as a powerful tool in predicting the ΔH of chemical reactions.
Measuring ΔH: Calorimetry and Hess’s Law
Delving into the realm of chemical reactions, one crucial parameter that unveils the energy dynamics is enthalpy change (ΔH). It quantifies the heat absorbed or released during a reaction, providing valuable insights into its nature and spontaneity.
Unveiling ΔH through Calorimetry
Calorimetry stands as a sophisticated experimental technique that meticulously measures the heat transfer associated with chemical reactions. Employing specialized equipment known as calorimeters, scientists can precisely determine the energy changes that accompany these transformations.
Diving into the Calorimeter’s Embrace
Calorimeters come in diverse forms, each meticulously designed to capture the heat flow during reactions. Some calorimeters, known as bomb calorimeters, trap the heat released in combustion reactions within a sealed environment, while solution calorimeters measure the temperature changes as reactants dissolve or neutralize.
Harnessing Hess’s Law: Unveiling the Intricate Web of Reactions
Beyond calorimetry, another pivotal concept in the realm of ΔH is Hess’s Law. This profound law unveils the remarkable fact that the total ΔH for a given reaction remains constant, regardless of the reaction pathway.
Deconstructing Hess’s Law
Hess’s Law asserts that the ΔH of a reaction is the sum of ΔH values for the individual steps that comprise the overall reaction. This principle empowers scientists to calculate ΔH for complex reactions by breaking them down into a series of simpler steps, each with a known ΔH.
Practical Applications of Hess’s Law
Hess’s Law finds invaluable applications in chemistry. It enables the calculation of ΔH for reactions that are difficult or impossible to measure directly. Additionally, it aids in the prediction of ΔH for reactions involving unstable intermediates. By piecing together the ΔH values of individual steps, chemists can gain a comprehensive understanding of the energy dynamics governing complex reactions.
Exothermic and Endothermic Reactions: The Heat Exchange Dance
In the realm of chemical reactions, we encounter two intriguing types: exothermic and endothermic reactions. These reactions are classified based on the enthalpy change (ΔH) that accompanies them. Enthalpy, in essence, measures the heat content of a system.
Exothermic Reactions: Heat on the Loose
Exothermic reactions are the generous ones in the chemical world. They release heat into their surroundings, making the universe a warmer place. This heat release is reflected in a negative ΔH value.
Imagine a cozy campfire on a chilly night. As logs burn, they react with oxygen to produce carbon dioxide and water vapor, releasing heat in the process. This heat warms you up, making you forget about the cold. Just like the campfire, exothermic reactions warm their surroundings by releasing heat.
Endothermic Reactions: Heat Seekers
Endothermic reactions, on the other hand, are heat seekers. They absorb heat from their surroundings to fuel the reaction, resulting in a positive ΔH value.
Think about the process of melting an ice cube. When you add heat to the ice, it absorbs energy to break apart its rigid structure, turning into liquid water. In the same way, endothermic reactions absorb heat from their surroundings to bring about chemical change.
The Spontaneity Saga
The sign of ΔH has a profound impact on the spontaneity of reactions. An exothermic reaction (ΔH < 0) is typically more spontaneous, meaning it occurs more readily and tends to favor the formation of products.
On the flip side, an endothermic reaction (ΔH > 0) is less spontaneous and may require an external energy input to initiate and proceed. It’s like pushing a car uphill; you need to apply force to overcome the resistance.
Calculating Enthalpy Change Using Standard Enthalpy of Formation
To calculate the enthalpy change (ΔH) of a chemical reaction, we often rely on standard enthalpy of formation values (ΔHf°). These values represent the enthalpy change when one mole of a compound is formed from its constituent elements in their standard states.
Let’s take a simple reaction as an example: the combustion of methane (CH4). The balanced chemical equation is:
CH4(g) + 2O2(g) → CO2(g) + 2H2O(l)
To calculate ΔH for this reaction, we need to look up the ΔHf° values for the reactants and products. These values can be found in tables or online databases.
ΔHf°(CH4) = -74.8 kJ/mol
ΔHf°(O2) = 0 kJ/mol (by definition)
ΔHf°(CO2) = -393.5 kJ/mol
ΔHf°(H2O) = -285.8 kJ/mol
Now, we can use the following equation to calculate ΔH:
ΔH = ΔHr° (products) - ΔHr° (reactants)
Substituting the values, we get:
ΔH = [(-393.5 kJ/mol) + (-285.8 kJ/mol)] - [(-74.8 kJ/mol) + (0 kJ/mol)]
ΔH = -683.1 kJ/mol
This negative ΔH value indicates that the combustion of methane is an exothermic reaction, meaning it releases heat to the surroundings.
By using standard enthalpy of formation values, we can easily calculate ΔH for a wide range of chemical reactions. This information is crucial for predicting the feasibility and spontaneity of reactions, as well as for designing and optimizing chemical processes.
Unveiling the Powers of Enthalpy Change (ΔH) in the Realm of Chemistry
Beyond its significance in understanding chemical reactions, understanding enthalpy change (ΔH) opens a gateway to a kaleidoscope of applications, empowering chemists to predict, optimize, and navigate the intricate world of molecular transformations.
- Predicting Reaction Feasibility and Spontaneity:
ΔH provides a crucial indicator of the feasibility and spontaneity of chemical reactions. Exothermic reactions (ΔH < 0), where energy is released, tend to occur spontaneously, while endothermic reactions (ΔH > 0), where energy is absorbed, typically require external energy input to proceed.
- Designing and Optimizing Chemical Processes:
The knowledge of ΔH is indispensable in designing and optimizing industrial chemical processes. By carefully manipulating the ΔH of reactions, chemists can increase product yield, minimize energy consumption, and enhance process efficiency.
- Comprehending Chemical Bonding and Energy Changes:
ΔH sheds light on the nature of chemical bonding and energy changes that accompany reactions. Exothermic reactions generally indicate stronger bond formation, while endothermic reactions suggest weaker bond formation or bond breaking. By analyzing ΔH, chemists gain insights into the energetic aspects of molecular rearrangements.
In the annals of chemistry, ΔH has proven to be an invaluable tool, empowering researchers to unravel the mysteries of chemical reactions, innovate groundbreaking processes, and deepen our understanding of the molecular world. It stands as a testament to the power of thermodynamics in shaping our comprehension of the chemical realm.