Mastering Carbon Bonds: Understanding Hybridization And Covalent Bonding
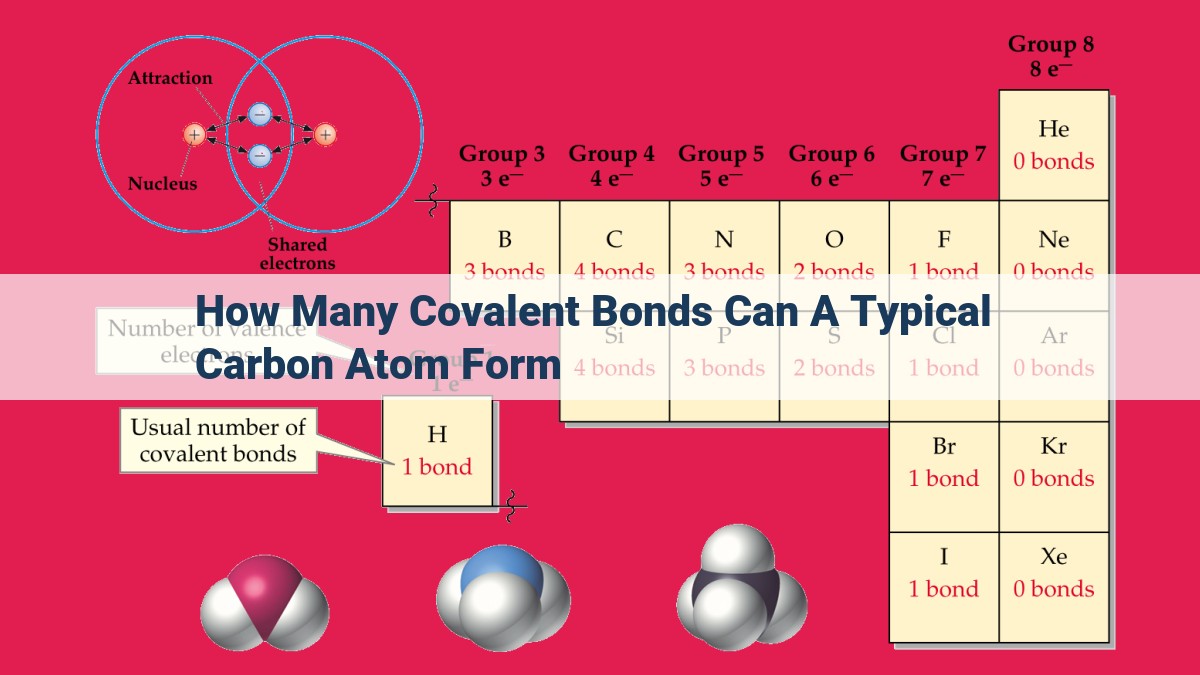
A typical carbon atom possesses four valence electrons, enabling it to form covalent bonds by sharing these electrons with other atoms. Depending on its hybridization state, carbon can form various types of covalent bonds: single (sp3), double (sp2), and triple (sp) bonds. The number of bonds formed is determined by the hybridization state, which dictates the number of unhybridized p-orbitals available for bond formation. For instance, in sp3 hybridization, carbon forms four single bonds; in sp2 hybridization, it forms three single bonds and one double bond; and in sp hybridization, it forms two single bonds and one triple bond.
Valence Electrons in Carbon Atoms
Imagine yourself as a chef preparing a delicious meal. Just like you need the right ingredients for your dish, atoms need specific components to form bonds and create molecules. These components are called valence electrons.
Carbon atoms, the building blocks of countless organic molecules, typically have four valence electrons. Think of them as four tiny magnets, ready to interact with other atoms. These valence electrons play a crucial role in chemical reactions, determining the types of bonds carbon can form.
Just as magnets attract or repel each other, valence electrons seek out partners to form stable bonds. This phenomenon is what holds atoms together, creating the vast array of compounds that make up our world.
Hybridization of Carbon Atom Orbitals: Unlocking the Versatile Chemistry of Life
In the realm of chemistry, understanding the behavior of atoms and their ability to form bonds is crucial for unraveling the secrets of molecular interactions. Carbon atoms, the building blocks of life, possess a unique characteristic that sets them apart: their ability to hybridize their atomic orbitals. This remarkable phenomenon shapes the geometry and energy levels of carbon’s orbitals, giving rise to the diverse array of molecules that make up our world.
Hybridization is the process by which atomic orbitals of different shapes and energies combine to form new hybrid orbitals with distinct properties. In the case of carbon, the hybridization of its 2s and 2p orbitals creates three primary types of hybrid orbitals: sp3, sp2, and sp. Each type of hybridization gives carbon specific geometrical arrangements and bonding capabilities.
sp3 Hybridization:
When one s orbital and three p orbitals hybridize, they form four equivalent sp3 hybrid orbitals. These hybrid orbitals are directed towards the four corners of a tetrahedron, resulting in a tetrahedral geometry. Molecules with sp3 hybridized carbon atoms exhibit a symmetrical structure and are nonpolar. Examples of sp3 hybridization include methane (CH4) and ethane (C2H6).
sp2 Hybridization:
In sp2 hybridization, one s orbital and two p orbitals combine to form three equivalent sp2 hybrid orbitals. These orbitals lie in a plane, forming a trigonal planar geometry. Molecules with sp2 hybridized carbon atoms are flat and have a double-bond character. Examples of sp2 hybridization include ethylene (C2H4) and benzene (C6H6).
sp Hybridization:
When one s orbital and one p orbital hybridize, they form two equivalent sp hybrid orbitals oriented linearly opposite each other. Molecules with sp hybridized carbon atoms have a linear geometry and exhibit triple-bond character. An example of sp hybridization is acetylene (C2H2).
The hybridization state of a carbon atom directly influences the number of covalent bonds it can form. sp3 hybridized carbon atoms can form four covalent bonds, sp2 hybridized carbon atoms can form three covalent bonds, and sp hybridized carbon atoms can form two covalent bonds. This concept underpins the vast array of organic molecules, which are characterized by their intricate bonding patterns and diverse properties.
Unveiling the Secrets of Single Covalent Bonds: How Carbon Atoms Forge Unbreakable Connections
Defining Covalent Bonds: A Tale of Shared Electrons
Imagine a dance party where atoms sway and mingle, eagerly exchanging their electrons like tiny, energetic partners. This intricate dance is known as covalent bonding, a process where atoms share electrons to achieve a state of perfect harmony. In the world of chemistry, carbon atoms, with their unparalleled versatility, reign supreme as masters of covalent bond formation.
How Carbon Forms Single Bonds: A Simple Yet Profound Embrace
When carbon atoms waltz together, they tend to share one pair of electrons, forming an inseparable bond known as a single covalent bond. Picture two carbon atoms, like graceful figures intertwining their fingers. This shared electron pair creates a strong and stable link between the atoms, holding them together like an unyielding embrace.
Properties of Single Covalent Bonds: Stability and Versatility
Single covalent bonds, like the foundation of a sturdy building, provide unmatched stability to molecules. They resist breaking under normal conditions, ensuring the integrity of molecular structures. Moreover, single bonds offer remarkable versatility, allowing carbon atoms to connect with a wide range of other atoms, including hydrogen, oxygen, and nitrogen. This versatility underpins the vast diversity of organic molecules that form the bedrock of life on Earth.
Examples of Single Covalent Bonds: Uncovering the Hidden Structure of Molecules
In the molecular realm, single covalent bonds play a crucial role in shaping the properties and behavior of countless substances. For instance, in the methane molecule (CH₄), four single bonds connect the central carbon atom to four hydrogen atoms, creating a symmetrical, tetrahedral structure. Similarly, in carbon dioxide (CO₂), two single bonds link the carbon atom to two oxygen atoms, forming a linear molecule that plays a vital role in our planet’s atmosphere.
Single covalent bonds, the basic building blocks of molecular architecture, hold immeasurable importance in the realm of chemistry. Carbon’s ability to form these bonds with exceptional stability and versatility empowers it to construct a myriad of organic molecules, the very foundation of life itself. From the intricate dance of carbon atoms to the countless molecules they shape, single covalent bonds orchestrate the symphony of chemical reactions and define the very fabric of our world.
Double Covalent Bonds: The Powerhouse of Carbon Chemistry
In the realm of carbon chemistry, double covalent bonds play a pivotal role, shaping the very essence of organic molecules. Let’s delve into the fascinating world of double bonds and uncover their profound significance.
When two carbon atoms share two pairs of valence electrons, they form a sturdy connection known as a double covalent bond. Unlike single bonds, double bonds exhibit unique properties and characteristics that endow organic molecules with remarkable stability and versatility.
The strength of a double bond lies in its two shared electron pairs, which create a concentrated electron density between the bonded atoms. This electron-rich zone, known as the pi bond, imparts exceptional rigidity to the bond. Consequently, double bonds exhibit shorter bond lengths and higher bond energies compared to single bonds.
Organic molecules often contain multiple double bonds, giving rise to conjugated systems. These systems possess alternating single and double bonds that facilitate the resonance of electrons, leading to enhanced stability and reduced chemical reactivity. Conjugated systems are found in countless organic compounds, including dyes, antioxidants, and vitamins.
The presence of double bonds also influences the geometry of molecules. Double bonds adopt a trigonal planar arrangement, where the bonded atoms and their neighboring atoms lie on the same plane. This geometry allows for cis-trans isomerism, a phenomenon where two identical groups can occupy either the same (cis) or opposite (trans) sides of the double bond.
In summary, double covalent bonds are the key to understanding the rich diversity and remarkable properties of organic molecules. From the stability of conjugated systems to the intricate geometry of molecules, double bonds play a crucial role in shaping the world of carbon chemistry. They are the unsung heroes that give organic molecules their unique identity and endless applications.
Triple Covalent Bonds: The Ultimate Carbon Connection
Beyond the realm of single and double bonds, the world of carbon chemistry unveils a fascinating phenomenon called triple bonds. A triple bond, as its name suggests, is a covalent bond formed when two atoms share three pairs of electrons instead of one or two.
In the case of carbon, this extraordinary bond forms when a carbon atom hybridizes its orbitals into the sp state. This hybridization results in three highly directional and elongated orbitals, each containing a single electron. When these three orbitals overlap with three orbitals from another atom, such as nitrogen or oxygen, they give rise to a triple bond.
Triple bonds are characterized by their exceptional strength and short bond length. The three shared electron pairs create a strong electrostatic attraction between the two atoms, holding them together with an unwavering grip. This strong bond imparts unique properties to molecules containing triple bonds, such as rigidity and stability.
Properties and Characteristics of Triple Bonds:
- Exceptional Strength: Triple bonds are the strongest type of covalent bond, resisting breaking even under extreme conditions.
- Short Bond Length: Due to the strong attraction between the three shared electron pairs, triple bonds have the shortest bond lengths among carbon-carbon bonds.
- Linear Geometry: The three highly directional sp orbitals involved in the triple bond force the atoms to adopt a linear arrangement.
- Polarizability: Triple bonds are less polarizable than single and double bonds, meaning they do not easily distort when subjected to external electric fields.
- High Energy: The formation of a triple bond requires a significant amount of energy compared to single or double bonds.
Triple bonds play a vital role in the architecture of many organic molecules, particularly those involved in biochemical processes. For instance, the triple bond in the acetylene molecule (C2H2) is essential for the production of many plastics and synthetic materials. Additionally, the triple bond in nitrogen gas (N2) makes it largely inert and abundant in the Earth’s atmosphere.
The formation of triple bonds in carbon chemistry is a testament to the versatility and complexity of this remarkable element. Understanding the nature and properties of triple bonds provides a deeper appreciation for the intricacies of molecular bonding and the boundless possibilities of carbon-based molecules.
The Variable Bonds of Carbon: Shaping the Fabric of Life
Carbon, the versatile building block of life, possesses a unique ability to form covalent bonds with itself and other elements, leading to the vast diversity of organic molecules that make up our world. At the heart of this versatility lies the concept of hybridization, a phenomenon that alters the orbitals of carbon atoms, dictating the number and types of bonds they can form.
Each carbon atom has four valence electrons, eager to engage in bonding adventures. When hybridized, these electrons rearrange themselves into new hybrid orbitals that differ in shape and energy levels. The three main types of hybridization are:
-
sp³ hybridization: Four equivalent sp³ hybrid orbitals form a tetrahedral geometry. This occurs when carbon is bonded to four other atoms, as in methane (CH₄).
-
sp² hybridization: Three sp² hybrid orbitals form a trigonal planar geometry. This occurs when carbon is bonded to three other atoms, as in ethylene (C₂H₄).
-
sp hybridization: Two sp hybrid orbitals form a linear geometry. This occurs when carbon is bonded to two other atoms, as in acetylene (C₂H₂).
The hybridization state of a carbon atom directly influences the number of covalent bonds it can form. In sp³ hybridization, each carbon can form four single bonds, as in methane. In sp² hybridization, it can form three single bonds or one double bond, as in ethylene. In sp hybridization, it can form two single bonds or one triple bond, as in acetylene.
Understanding the number of covalent bonds formed by carbon is crucial for comprehending the structure and properties of organic molecules. This knowledge paves the way for further exploration of the fascinating world of carbon chemistry, where atoms dance together in countless configurations, giving rise to the intricate tapestry of life.