Magnetism: A Guide To The Fundamental Force That Shapes The World
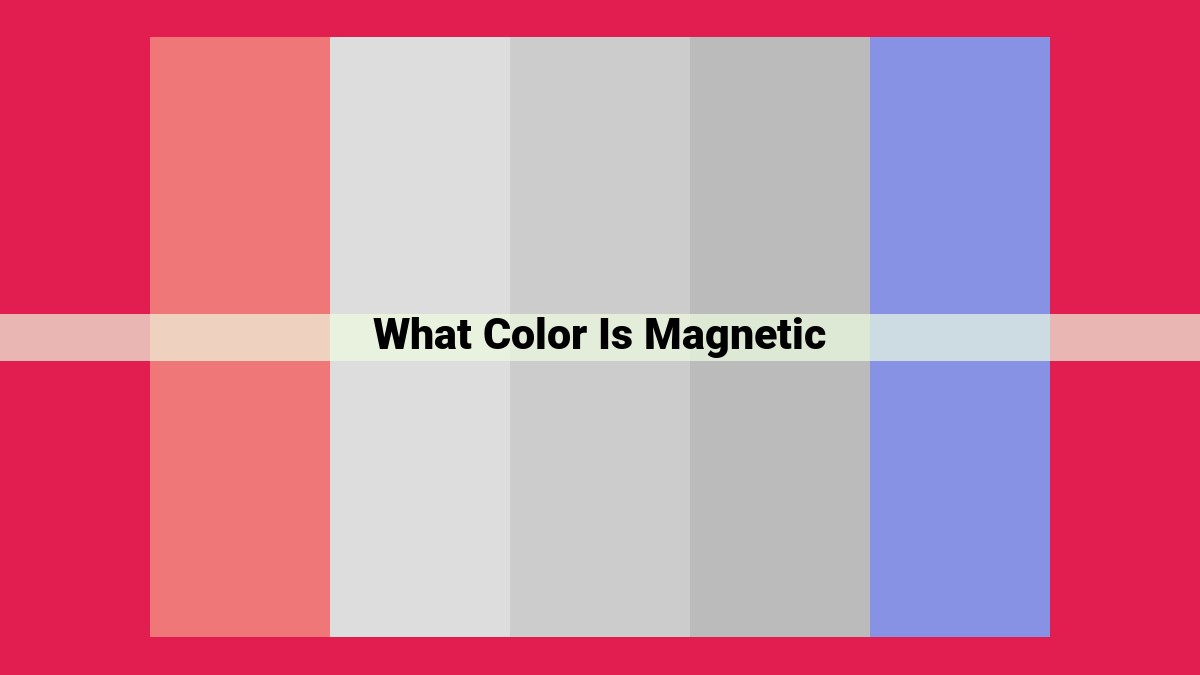
- What is Magnetism?
- Define magnetism as a fundamental force of nature.
- Explain its role in various phenomena and applications.
Magnetism is a fundamental force of nature that attracts or repels certain materials. It is responsible for the attraction between magnets and magnetic materials, and it plays a role in a wide range of phenomena, including the Earth’s magnetic field, electric motors, and magnetic resonance imaging (MRI).
What is Magnetism?
- Define magnetism as a fundamental force of nature.
- Explain its role in various phenomena and applications.
What is Magnetism?
Magnetism, an alluring force of nature, has captured the imagination and driven scientific inquiry for centuries. It’s an invisible power that holds sway over our world, from the smallest particles to the grandest celestial bodies.
The Magnetism Chronicles
Magnetism was first discovered in ancient Greece, where philosophers observed the peculiar behavior of certain stones called lodestones. These stones possessed the uncanny ability to attract iron objects. This phenomenon sparked curiosity and laid the foundation for the study of magnetism.
A Force of Nature
Magnetism is one of the four fundamental forces of nature, alongside gravity, the strong force, and the weak force. It acts between magnetic materials, aligning their magnetic moments and influencing their behavior. Magnetism plays a pivotal role in a vast array of natural phenomena, from the majestic auroras that dance across the night sky to the intricate dance of atoms within molecules.
Unveiling the Magnetic Universe
Magnetism finds practical applications in countless technologies that shape our modern world. Electric motors, generators, MRI scanners, and compasses all rely on the principles of magnetism to function. In the realm of space exploration, magnetic fields shield spacecraft from harmful radiation and enable navigation through interplanetary voyages.
By unraveling the mysteries of magnetism, we gain a deeper understanding of our universe and unlock the potential for groundbreaking innovations. From the ancient Greeks to present-day scientists, the allure of magnetism continues to inspire and captivate, promising new discoveries and shaping the future of our technological endeavors.
Magnetic Field Lines: Visualizing the Invisible
Imagine a world where you could see the invisible. In the realm of magnetism, we have exactly that: magnetic field lines. These ethereal lines help us visualize and comprehend the enigmatic force of magnetism.
Mapping the Magnetic Force
Magnetic field lines are imaginary pathways that map the direction and strength of a magnetic field. Picture an invisible compass needle suspended in space, always aligning itself with the local magnetic field. The direction of the field line at any point indicates the direction the needle would point. Just as a stream of water flows in a certain direction, so too do magnetic field lines flow along the path of least resistance.
Origins and Infinity
These field lines originate from magnetic poles, analogous to the positive and negative terminals of a battery. Magnetic poles are regions where the magnetic field is strongest. From these poles, the field lines extend indefinitely, forming a continuous network throughout space. It’s as if the magnetic poles are emitting an invisible force that permeates the universe.
Magnetic Field Intensity
The intensity of a magnetic field, or its strength, is represented by the density of the field lines. Where field lines are densely packed, the magnetic field is stronger. Imagine a crowded highway during rush hour, where each car represents a magnetic field line. The more cars there are, the more intense the traffic, and the stronger the field.
Magnetic Field Strength: Unveiling the Force Behind Magnetic Phenomena
In the realm of magnetism, magnetic field strength emerges as a key concept, shedding light on the intensity and direction of the invisible forces at play. It serves as a metric for quantifying the strength of a magnetic field at a particular point in space. Imagine a tapestry woven with invisible threads of magnetic force, with the strength of these threads varying from one point to another. Magnetic field strength serves as the measuring tape for these threads, painting a vivid picture of the magnetic landscape.
Key to understanding magnetic field strength is its intimate relationship with magnetic field lines. These imaginary lines trace the path of magnetic forces, revealing their direction and intensity. The strength of the magnetic field is directly proportional to the density of these lines, with regions of higher line density indicating stronger magnetic forces.
Magnetic field strength is also intricately connected to two material properties: magnetic susceptibility and magnetic permeability. Magnetic susceptibility measures the ease with which a material can be magnetized. Materials with high susceptibility willingly respond to the presence of magnetic fields, while those with low susceptibility resist their influence. Magnetic permeability, on the other hand, quantifies a material’s ability to conduct magnetic fields. High-permeability materials serve as efficient channels for magnetic forces, while low-permeability materials offer resistance to their flow.
The interplay of these properties profoundly influences the strength of magnetic fields within materials. Magnetic field strength is directly proportional to the product of susceptibility and permeability. Materials with both high susceptibility and high permeability amplify magnetic fields, while those with low susceptibility and low permeability diminish their strength.
Understanding magnetic field strength is paramount in unraveling the behavior of magnetic systems. It governs the force exerted on moving charges, the interaction between magnets, and the functioning of countless electromagnetic devices. From the colossal magnets employed in MRI machines to the miniature motors that power our everyday gadgets, magnetic field strength plays a crucial role in shaping their performance and applications.
Magnetic Poles: The Heart of Magnetism
Imagine magnets as two ends of an invisible force. Just like a battery has positive and negative terminals, magnets have magnetic poles – North and South. These poles are the focal points of a magnetic field, where the field strength is at its peak.
Magnetic field lines, like invisible threads, flow out of the North pole and converge at the South pole. These lines represent the direction and strength of the magnetic field. The magnetic flux, a measure of the total magnetic field flowing through a surface, is highest at the poles.
Magnetic poles play a vital role in various magnetic phenomena. They attract and repel other magnets, aligning their fields to form chains or patterns. This magnetic attraction is the basis for many everyday devices, from compasses to electric motors.
Just as battery terminals create an electric field, magnetic poles generate a magnetic field. The magnetic field strength is strongest near the poles and weakens as you move away. This field extends indefinitely, creating an invisible force that interacts with other magnetic materials.
The analogy between magnetic poles and electric battery terminals is a helpful way to visualize their function. Both poles represent points of concentrated force, creating a field that influences nearby objects. Understanding magnetic poles is essential for comprehending the nature of magnetism and its countless applications in our world.
Magnetic Susceptibility: Unveiling a Material’s Magnetic Charm
In the realm of magnetism, materials exhibit a fascinating diversity in their response to magnetic fields. Some materials, like iron, eagerly attract and become magnetized, while others remain indifferent, like plastic. This intriguing behavior can be explained by a property known as magnetic susceptibility.
Defining Magnetic Susceptibility
Magnetic susceptibility is a measure of how easily a material can be magnetized when subjected to an external magnetic field. It quantifies the degree to which a material acquires a magnetic moment, an intrinsic property that represents the object’s magnetic strength.
The Role of Magnetic Susceptibility
Magnetic susceptibility plays a crucial role in determining the induced magnetic field within a material. When an external magnetic field is applied, susceptible materials respond by developing their own magnetic field, aligning themselves with the external field. The strength of this induced magnetic field is directly proportional to the magnetic susceptibility of the material.
Susceptibility and Magnetic Properties
Magnetic susceptibility is closely related to other magnetic properties, including magnetic field strength and magnetic permeability. Magnetic field strength measures the intensity of the magnetic field at a given point, while magnetic permeability represents a material’s ability to conduct magnetic fields. Susceptibility, permeability, and field strength together govern the magnetic behavior of materials.
Materials with Different Susceptibilities
Different materials exhibit varying degrees of magnetic susceptibility:
- Diamagnetic Materials: These materials (e.g., water, gold, copper) have a negative magnetic susceptibility. They weakly oppose external magnetic fields, causing a slight decrease in the magnetic field within the material.
- Paramagnetic Materials: Materials with a positive magnetic susceptibility (e.g., aluminum, oxygen, sodium) enhance the external magnetic field by creating a small induced field in the same direction.
- Ferromagnetic Materials: These materials (e.g., iron, nickel, cobalt) possess a very high magnetic susceptibility, allowing them to become strongly magnetized in the presence of an external field.
Understanding magnetic susceptibility is essential for comprehending the diverse magnetic behaviors observed in materials and their applications in various technologies, such as magnets, sensors, and magnetic resonance imaging (MRI).
Magnetic Permeability
- Define magnetic permeability as a measure of material’s ability to conduct magnetic fields.
- Explain related concepts: magnetic susceptibility, magnetic flux, and magnetic field strength.
- Discuss how permeability affects magnetic field penetration in a material.
Magnetic Permeability: Understanding the Ability of Materials to Conduct Magnetic Fields
In the realm of magnetism, each material exhibits a unique ability to conduct magnetic fields. This distinctive characteristic is quantified by a parameter known as magnetic permeability.
Permeability, symbolized by the Greek letter mu (ยต), is a dimensionless quantity that reflects how easily a material allows magnetic fields to penetrate its structure. Imagine a material as a mesh of tiny magnetic dipoles, each with a preference to align with an applied magnetic field. Permeability measures how readily these dipoles respond to the field, determining the intensity and distribution of the magnetic field within the material.
The higher the permeability of a material, the more effectively it can concentrate and transmit magnetic flux. Such materials are known as ferromagnetic and include substances like iron, nickel, and cobalt. Conversely, materials with low permeability are termed diamagnetic and exhibit a weaker response to magnetic fields. Air and water fall under this category.
Permeability is not only crucial for understanding magnetic phenomena but also has practical implications in engineering applications. High-permeability materials are often used in magnetic circuits to enhance magnetic field strength and reduce energy losses. For instance, in transformers, highly permeable iron cores are employed to maximize magnetic flux, improving energy efficiency.
On the other hand, materials with low permeability find applications in shielding sensitive electronic devices from external magnetic fields. Such materials create barriers that impede the penetration of unwanted magnetic disturbances, ensuring the proper functioning of instruments in environments with strong magnetic interference.
In summary, magnetic permeability is an essential parameter that governs the interaction between magnetic fields and materials. It determines the strength and distribution of magnetic fields within materials, and its understanding is critical for optimizing magnetic circuits, shielding devices, and advancing our understanding of magnetic phenomena.
Magnetic Flux: Understanding the Flow of Magnetic Fields
In the realm of magnetism, magnetic flux plays a crucial role in quantifying the movement of magnetic fields. It represents the total amount of magnetic field that penetrates a particular surface. Think of it as a measure of the magnetic field’s “flow rate.”
Magnetic flux is directly influenced by several key concepts:
- Magnetic poles: These are the areas where magnetic fields are strongest.
- Magnetic permeability: This property describes a material’s ability to conduct magnetic fields.
- Magnetic field strength: This measures the strength of the magnetic field at a specific point.
Understanding magnetic flux allows us to gain valuable insights into the behavior of magnetic fields within a specified region. For instance, the higher the magnetic flux, the stronger the magnetic field passing through that surface. This makes it essential for analyzing magnetic circuits and designing electromagnetic devices.
Magnetic flux is often symbolized by the Greek letter ฮฆ (phi) and is measured in units called webers (Wb). It is calculated by integrating the magnetic field strength over the given surface area. By understanding magnetic flux, we can gain a deeper comprehension of the complex world of magnetism and its numerous applications.