Light-Dependent Photosynthesis: Converting Sunlight Into Chemical Energy For Plant Life
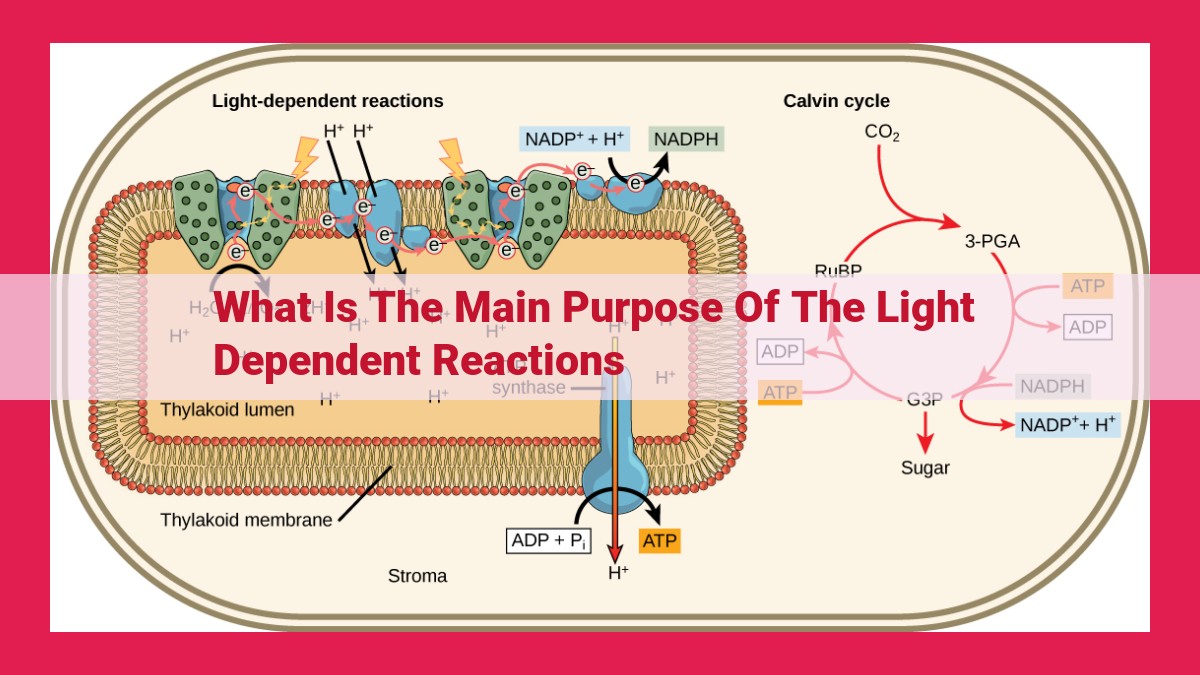
The primary purpose of the light-dependent reactions in photosynthesis is to capture light energy from the sun and convert it into chemical energy stored in ATP and NADPH. Chlorophyll and antenna complexes absorb and channel light energy to Photosystem I and Photosystem II, which capture different wavelengths of light. The electron transport chain transfers electrons, releasing energy used to generate a proton gradient for ATP synthesis and reduce electron carriers for NADPH production. These energy molecules (ATP and NADPH) are essential for the Calvin cycle, which uses the electrons to reduce CO2 into glucose. Additionally, the light-dependent reactions release oxygen as a byproduct through the splitting of water molecules.
Explain the role of chlorophyll and antenna complexes in absorbing and channeling light energy.
Capturing Light Energy: The Symphony of Chlorophyll and Antenna Complexes
In the captivating world of photosynthesis, the journey begins with the capturing of light energy from the sun’s golden rays. Here, two key players take center stage: chlorophyll and antenna complexes.
Chlorophyll, the mastermind behind photosynthesis, is a pigment that resides within the chloroplasts of plant cells. It possesses an uncanny ability to absorb light energy in the blue and red spectrums, but the story doesn’t end there. To maximize light absorption, chlorophyll teams up with antenna complexes, acting as a synchronized dance troupe.
Antenna complexes are large, colorful proteins that surround chlorophyll molecules. They act as light-harvesting machines, capturing photons of light in a wide range of wavelengths, including those that chlorophyll misses. Once captured, these photons are funneled and channeled towards chlorophyll, like a symphony of music flowing to the conductor. Through this harmonious collaboration, plants have evolved a remarkable strategy to efficiently capture the sun’s energy, fueling the very foundation of life on our planet.
Photosynthesis: The Vital Process of Capturing Sunlight and Converting it into Energy
1. Capturing Light Energy from the Sun
Like an orchestra tuning their instruments, chlorophyll and antenna complexes within plant cells diligently absorb the sun’s radiant energy. These pigments act as nature’s light collectors, orchestrating a symphony of light absorption and energy transfer.
Within this intricate molecular dance, two key players emerge: Photosystem I and Photosystem II. These protein complexes serve as specialized receptors, each attuned to specific wavelengths of light.
Photosystem II stands as the initial gatekeeper, capturing high-energy photons from the blue and red portions of the spectrum. Photosystem I, a complementary ensemble, intercepts the remaining energy carried by longer-wavelength orange and far-red light.
Through this harmonious interplay, Photosystem I and Photosystem II work in concert, capturing the full spectrum of sunlight and channeling its energy into the intricate machinery of photosynthesis.
Discuss the electron transport chain and how it transfers electrons, releasing energy.
Harnessing the Sun’s Energy: The Intricate Alchemy of Photosynthesis
In the tapestry of life, photosynthesis stands as a grand masterpiece, where sunlight, the lifeblood of our planet, is transformed into the fuel that sustains all living things. At the heart of this remarkable process lies the electron transport chain, an intricate network that orchestrates the transfer of electrons, releasing the energy that powers the creation of life’s essential building blocks.
Imagine an intricate dance of tiny particles, where chlorophyll captures the golden rays of sunlight, channeling their energy into the dance floor of the electron transport chain. With each graceful step, electrons pirouette through a series of protein complexes, shedding their energy like shimmering sequins, creating a cascade of power.
This energy surge pumps protons across a membrane, akin to building up a mighty reservoir of electrical potential. As these protons tumble back down their electrochemical gradient, they drive the synthesis of ATP, the cellular currency of energy, providing the fuel for countless biological processes.
Along this electron-laden path, NADPH is forged, a crucial electron carrier destined for the Calvin cycle, where carbon dioxide is transformed into the sugars that nourish every living organism.
This intricate symphony of electron transfer lies at the foundation of photosynthesis, the life-giving process that sustains our planet’s vibrant tapestry. It is a testament to the remarkable ingenuity of nature, a masterpiece of molecular engineering that deserves our deepest appreciation and awe.
Unveiling the Secret of Energy Conversion in Photosynthesis: The Proton Gradient and ATP Synthesis
Photosynthesis, the lifeblood of our planet, is a captivating dance of light and chemistry. In this intricate ballet, plants harness the sun’s energy to transform carbon dioxide and water into glucose, the fuel for all life. But beneath the surface of this elegant process lies a hidden symphony, where a proton gradient orchestrates the very essence of life – the creation of ATP, the cellular energy currency.
As sunlight strikes the chlorophyll molecules nestled within the plant’s cells, it sets off a chain reaction, exhilaratingly referred to as the electron transport chain. This electrifying journey of electrons releases energy, carefully funneled into pumping protons (H+) across a membrane, creating an electrochemical gradient.
This gradient, like a miniature hydroelectric dam, is the driving force behind the breathtaking creation of ATP. As protons rush back through a channel, known as ATP synthase, the spinning turbine-like motion powers the synthesis of ATP from ADP. ATP, the cellular workhorse, is the energy that energizes all life’s processes, from cell division to the flicker of a butterfly’s wings.
Without this proton gradient, the flow of energy would seize, and life on Earth would grind to a halt. It is a testament to the exquisite design of nature, where the most fundamental of processes are orchestrated with such elegance and efficiency.
Photosynthesis: The Symphony of Life on Earth
In the realm of life, there exists a remarkable harmony between the sun’s energy and the Earth’s living organisms. This harmonious dance is known as photosynthesis, where plants, algae, and certain bacteria transform light energy into the chemical energy that sustains all life.
The process of photosynthesis is a captivating journey that unfolds in five distinct steps:
- Capturing Light Energy from the Sun:
Chlorophyll, the green pigment that adorns leaves, acts as a maestro in absorbing light energy. It orchestrates a team of antenna complexes that channel this energy to two crucial components: Photosystem I and Photosystem II. Each photosystem captures specific wavelengths of light, like a pair of musical notes, resonating with the sun’s melody.
- Converting Light Energy into Chemical Energy:
The absorbed light energy sets electrons on a journey through an intricate electron transport chain. These electrons, like a vibrant stream of notes, release energy as they cascade down this cellular energy ladder. This energy drives the creation of a proton gradient, an energetic barrier that powers the generation of ATP, the universal currency of energy in cells.
- Producing ATP and NADPH:
ATP, a molecule brimming with energy, is a key player in cellular processes. Its formation marks the climax of the photosynthetic symphony. Another crucial molecule, NADPH, also emerges from this energy-conversion process, carrying electrons that will fuel the Calvin cycle, the stage for carbon fixation.
- Providing Electrons for the Calvin Cycle:
NADPH and its cohort of electrons embark on a circuitous electron transfer pathway, passing through a quartet of musical instruments—ferredoxin, plastocyanin, cytochrome b6f, and cytochrome c. This electron dance culminates in the reduction of NADP+, a molecule ready to donate electrons to the Calvin cycle, the photosynthetic stage where carbon is incorporated into organic molecules.
- Generating Oxygen as a Byproduct:
As the photosynthetic symphony reaches its finale, water molecules are split apart by a remarkable enzyme complex. Oxygen, a byproduct of this process, is released into the atmosphere, a life-sustaining melody that sustains Earth’s ecosystems. The electrons from water molecules join the electron transport chain, fueling the production of ATP and NADPH, the driving forces of photosynthesis.
Explain the role of glycolysis, the Krebs cycle, and oxidative phosphorylation in generating ATP.
The Extraordinary Journey of Photosynthesis: From Sunlight to Biochemical Energy
Capturing the Sun’s Radiance
Photosynthesis begins with the capture of light energy by chlorophyll, a green pigment found in plant cells. Light energy is absorbed by antenna complexes, which channel it to Photosystem I and Photosystem II. These photosystems capture different wavelengths of light, initiating the conversion of light energy into chemical energy.
Transforming Light into Chemical Wealth
The electron transport chain is a critical player in photosynthesis. It facilitates the transfer of electrons from Photosystem II to Photosystem I. As electrons travel through the chain, their energy is released and used to pump protons across a membrane, creating a proton gradient. This gradient drives the synthesis of ATP, an energy-carrying molecule essential for cell function.
Generating the Powerhouse Molecules
ATP and NADPH are the powerhouses of photosynthesis. ATP is generated through a complex series of reactions involving glycolysis, the Krebs cycle, and oxidative phosphorylation. Glycolysis breaks down glucose into pyruvate, which is then fed into the Krebs cycle to produce NADH and FADH2. These molecules are essential for driving oxidative phosphorylation, the final stage of ATP production.
Fueling the Calvin Cycle
NADPH and ATP provide the electrons and energy necessary for the Calvin cycle. This cycle converts carbon dioxide into glucose, the primary energy source for plants. Electrons from Photosystem I are transferred through a series of molecules, including ferredoxin, plastocyanin, cytochrome b6f, and cytochrome c, before ultimately reducing NADP+ in the Calvin cycle.
Releasing Oxygen as a Gift
An intriguing byproduct of photosynthesis is the release of oxygen. The splitting of water molecules by the water-splitting complex and the oxygen-evolving complex generates electrons that drive photosynthesis while releasing oxygen into the atmosphere.
The Powerhouse of Photosynthesis: NADH and FADH2 in Oxidative Phosphorylation
Imagine a bustling city, where energy flows like a river. In the heart of this city, two powerhouses stand tall: NADH and FADH2. These molecular giants play a crucial role in driving oxidative phosphorylation, the process that generates most of the city’s energy currency: ATP.
ATP, the lifeblood of photosynthesis, is like the fuel that powers the cellular machinery. It’s used for everything from synthesizing sugars to transporting molecules. And NADH and FADH2 are the key to unlocking this vast reservoir of energy.
As sunlight pours into the city, it’s captured by chlorophyll and antenna complexes. This energy is then funneled into photosystems I and II, which split water molecules and release electrons. These electrons flow down an electron transport chain, releasing energy at each step.
NADH and FADH2 are like electron carriers, picking up these high-energy electrons and passing them along the chain. As the electrons move, the energy they release is used to pump protons across a membrane. This creates a proton gradient, which is like a battery, storing energy.
The powerhouse of oxidative phosphorylation, ATP synthase, harnesses this energy to generate ATP. As protons flow back across the membrane, through ATP synthase, they drive the synthesis of ATP from ADP and inorganic phosphate.
Without NADH and FADH2, the electron transport chain would grind to a halt, and the city would be devoid of energy. These tiny molecules are the unsung heroes, driving the production of ATP and fueling the vibrant life within the photosynthetic cell.
Providing Electrons for the Calvin Cycle: A Journey through Electron Pathways
In the intricate tapestry of photosynthesis, capturing light energy is just the first step. To harness this energy and transform it into life-sustaining molecules, a complex electron-transfer pathway comes into play. Like a relay race, electrons embark on a journey, passing through a series of molecular intermediaries, orchestrating essential reactions.
One key player in this electron relay is ferredoxin, a small protein that acts as an electron carrier. Once excited by electrons from photosystem I, ferredoxin passes them onto the mobile plastocyanin. This small, blue protein then journeys to cytochrome b6f, a protein complex that facilitates the transfer of electrons between the cytochrome chain and plastocyanin.
The final step in this relay involves cytochrome c, an electron carrier that donates the electrons to photosystem II. Energized by the electron transfer, photosystem II splits water molecules, releasing oxygen as a byproduct. This process, known as water splitting, fuels the continuous supply of electrons for photosynthesis.
Throughout this electron transfer pathway, the energy released is harnessed to create a proton gradient, a key driving force behind ATP synthesis. Furthermore, the reduction of NADP+ in the Calvin cycle relies on electrons from photosystem I, completing the intricate dance of photosynthesis.
Explain how electrons from photosystem I are used to reduce NADP+ in the Calvin cycle.
Harnessing Sunlight: A Journey of Energy Conversion in Photosynthesis
In the vibrant tapestry of life, photosynthesis plays a pivotal role, unlocking the secrets of transforming sunlight into chemical energy that sustains our planet. Join us on a journey into the intricate dance of light and life, where we uncover the remarkable processes that make photosynthesis possible.
Step 1: Capturing the Sun’s Radiance
Like a symphony of colors, chlorophyll molecules and antenna complexes orchestrate the absorption of light energy from the sun’s rays. These pigments act as the gateway to photosynthesis, channeling the energy into specialized structures called Photosystem I and Photosystem II.
Step 2: Energy Conversion Central
Within these photosystems, a chain of electron carriers embarks on a transformative dance, releasing energy as they pass from one to another. This energy is harnessed to pump protons across a membrane, creating a proton gradient. This gradient serves as the driving force for the synthesis of ATP, the universal currency of energy in cells.
Step 3: Electrons for the Calvin Cycle
A meticulous electron relay system, involving ferredoxin, plastocyanin, cytochrome b6f, and cytochrome c, orchestrates the flow of electrons from Photosystem I. These electrons ultimately reduce NADP+ molecules, creating NADPH, which is an essential electron donor for the Calvin cycle.
Step 4: The Calvin Cycle: Carbon Fixation
The Calvin cycle, a biochemical masterpiece, utilizes the electrons from NADPH to reduce carbon dioxide into sugars. Carbon dioxide is the building block of carbohydrates, the fundamental energy source for most organisms.
Step 5: Oxygen as a Byproduct
As a side effect of photosynthesis, water molecules are split, releasing oxygen into the atmosphere. This oxygen is an essential byproduct, sustaining the very breath of life on Earth.
Ecological Significance
Photosynthesis is more than just a scientific process; it’s the foundation of our ecological balance. As the primary producer in food chains, plants harness the sun’s energy to create the food that nourishes all life forms. Moreover, photosynthesis is a crucial regulator of Earth’s atmosphere, absorbing carbon dioxide and releasing oxygen, maintaining the delicate balance of our planet.
The Power of Photosynthesis: Untangling the Mystery of Oxygen Production
Photosynthesis is the magical process by which plants transform sunlight into the energy that fuels our planet. At the heart of this extraordinary dance lies the water-splitting complex, an intricate machinery responsible for unleashing the life-giving oxygen we breathe.
Nestled within the thylakoid membranes of chloroplasts, the water-splitting complex is an ensemble of proteins and chlorophyll molecules that holds the key to oxygen evolution. This complex initiates the process by absorbing an unprecedented amount of light energy, which it channels to a specialized protein called the oxygen-evolving complex.
The oxygen-evolving complex is a dynamic assembly of four manganese ions and a calcium ion. This intricate arrangement allows it to capture electrons from water molecules, breaking the covalent bonds that hold them together. With the water molecules dismantled, the oxygen atoms are liberated, combining to form the oxygen gas that we rely on for life.
As electrons are extracted from water, they embark on a remarkable journey through the electron transport chain, releasing energy that drives the synthesis of ATP and NADPH. These energy-rich molecules serve as the fuel for other crucial cellular processes.
The evolution of oxygen by photosynthesis is not merely a byproduct but an essential foundation for life on Earth. Without this vital process, the atmosphere would be devoid of oxygen, rendering it uninhabitable for complex life forms. The water-splitting complex, therefore, stands as a testament to the intricate interdependence of life and the power of nature’s grand design.
Photosynthesis: Harnessing the Sun’s Energy
The captivating journey of photosynthesis begins with the sun’s glorious rays, which are eagerly embraced by chlorophyll and its antenna complexes. These vibrant molecules act as energy absorbers, channeling the light’s radiance into the heart of the plant cell.
As the light energy flows onward, it encounters two crucial photosystems, known as Photosystem I and Photosystem II. Each photosystem is adept at capturing specific wavelengths of light, ensuring that the full spectrum of solar energy is utilized. This symphony of light absorption sets the stage for the transformation of light energy into chemical energy.
Venturing further into the photosynthetic realm, we encounter the intricate electron transport chain, where electrons embark on a lively dance, releasing bursts of energy as they traverse along this molecular pathway. This energetic dance fuels the formation of a proton gradient, a powerhouse that drives the synthesis of ATP, the primary energy currency of the cell.
Concurrently, NADPH is forged, a vital electron carrier that plays a pivotal role in the Calvin cycle, the photosynthetic factory that converts carbon dioxide into life-sustaining sugars. The electron transport chain orchestrates this symphony of energy transfer, ensuring a continuous supply of ATP and NADPH, the fuel that powers the cell’s metabolic processes.
As the photosynthetic engine hums along, a remarkable byproduct emerges: oxygen. This vital element is released as a consequence of the water-splitting complex splitting water molecules. The oxygen-evolving complex, a molecular maestro, orchestrates this process, releasing oxygen into the atmosphere, a vital life-giving force for our planet.
The story of photosynthesis is a testament to nature’s ingenuity, a process that harnesses the sun’s energy to sustain life on Earth. From the dance of electrons to the release of oxygen, every step in this intricate process underscores the remarkable interconnectedness of life and the boundless energy of the sun.
The Crucial Role of Plastocyanin in Photosynthesis
In the realm of photosynthesis, a molecular dance takes place, fueled by the sun’s radiant energy. Plastocyanin, a copper-containing protein, plays a pivotal role in this intricate symphony, facilitating the transfer of electrons between two key components: the water-splitting complex and Photosystem II.
The water-splitting complex, located within the thylakoid membrane, is a molecular machinery responsible for the liberation of electrons from water molecules. These electrons embark on a journey, first encountered by plastocyanin. Acting as a molecular bridge, plastocyanin captures these electrons and swiftly transports them to Photosystem II.
Photosystem II, situated on the thylakoid membrane, utilizes these transported electrons to generate an electrochemical gradient across the membrane, driving the production of ATP (adenosine triphosphate), the energy currency of cells.
Without plastocyanin, the electron transfer chain would be disrupted, impairing the generation of ATP and the entire photosynthetic process. It is through this critical role that plastocyanin ensures the seamless flow of electrons, underpinning the production of food, oxygen, and energy for life on Earth.