Ionic Bonding: The Force Behind Stable Table Salt Crystals
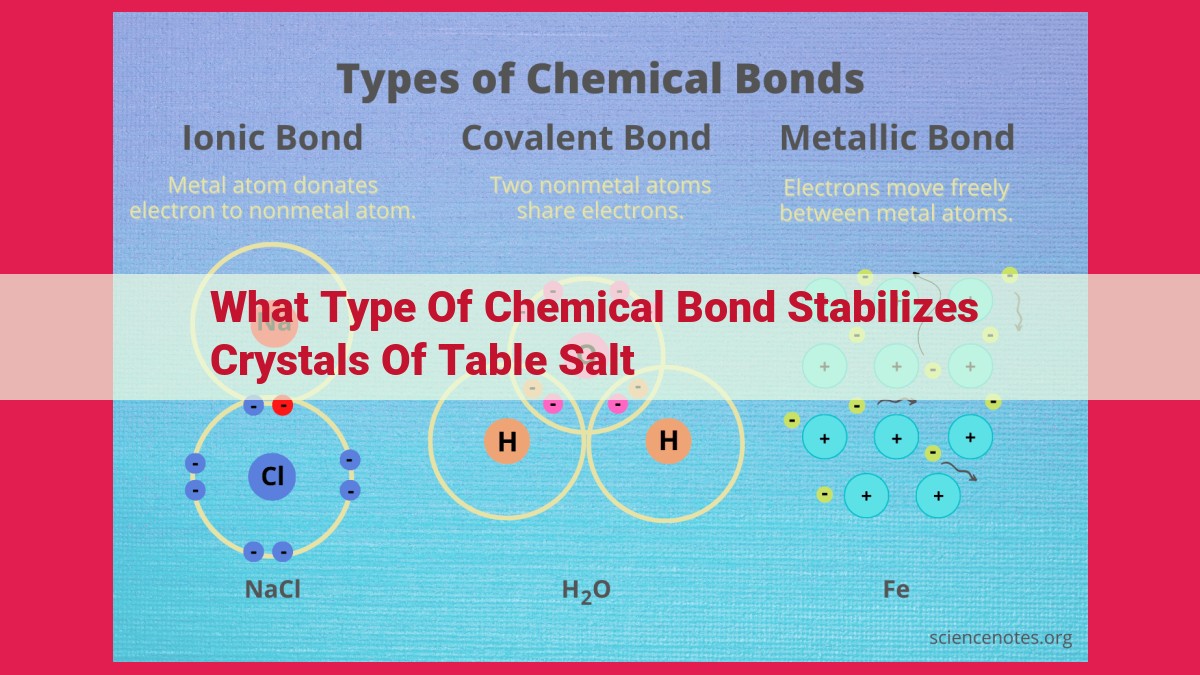
Ionic bonding stabilizes table salt crystals. This bond forms when electrons transfer from one atom to another, creating oppositely charged ions. The electrostatic attraction between these ions holds the crystal together. In table salt (NaCl), sodium loses an electron to chlorine, forming Na+ and Cl- ions. The strong electrostatic attraction between these ions contributes to the stability and high melting point of table salt crystals.
Ionic Bonding and Crystal Stability: The Bedrock of Matter
The world we live in is composed of an intricate tapestry of substances, each with unique properties and characteristics. At the heart of these properties lies the fundamental concept of ionic bonding, an invisible force that orchestrates the stability of countless materials, from the salt we sprinkle on our food to the rocks that form the Earth’s crust.
The Essence of Ionic Bonding
Ionic bonding, as its name suggests, is an electrostatic attraction between two oppositely charged ions. Ions themselves are atoms or molecules that have gained or lost electrons, leaving them with a net electrical charge. When a metal atom loses one or more electrons, it becomes a cation, while a non-metal atom that gains electrons becomes an anion.
Formation of Ionic Compounds
The formation of ionic compounds is a captivating process driven by electrostatic attraction. When a metal and a non-metal react, the metal atoms readily give up their outermost electrons, while the non-metal atoms eagerly accept them. This transfer of electrons creates an imbalance of charges, resulting in the formation of oppositely charged ions. These ions, like magnets with opposing polarities, are irresistibly drawn to each other, forming a stable ionic compound.
Ionic Crystals: A World of Order
The story of ionic bonding doesn’t end with the formation of ions. Once these charged particles have united, they arrange themselves in a highly organized, three-dimensional structure known as an ionic crystal. This crystalline architecture is a testament to the strong electrostatic forces that bind the ions together, resulting in a remarkable stability that gives ionic crystals their characteristic hardness and brittleness.
Ionic bonding, the invisible force that governs the stability of countless substances, is a captivating tale of electron transfer and electrostatic attraction. From the salt we season our food with to the rocks that form the very foundation of our planet, ionic bonding plays a pivotal role in shaping the world we perceive. By understanding its fundamental principles, we gain a deeper appreciation for the unseen forces that orchestrate the world around us.
Electrostatic Attraction and Ionization: The Driving Forces Behind Ionic Bonding
In the realm of chemistry, ionic bonding, a powerful force, holds atoms together to form ionic compounds, the building blocks of many everyday substances. Understanding the fundamental principles of electrostatic attraction and ionization is crucial to unraveling the secrets of these fascinating compounds.
Electrostatic Attraction: The Glue of Ions
Imagine oppositely charged particles drawn together like magnets: this is electrostatic attraction, the driving force behind ionic bond formation. When an atom donates or accepts electrons, it gains or loses an electrical charge, transforming it into an ion. These charged ions experience an intense attraction, forming the foundation of ionic bonds.
Ionization: The Birth of Ions
The process of ionization is the catalyst for ionic bond formation. When an atom loses one or more electrons, it becomes a cation, bearing a positive charge. Conversely, an atom that gains electrons transforms into an anion, carrying a negative charge.
Formation of Cations and Anions
Metals tend to lose electrons easily, forming cations. For example, one sodium atom (Na) readily gives up an electron, becoming a sodium cation (Na+). Nonmetals, on the other hand, have a strong affinity for electrons and readily accept them, forming anions. A chlorine atom (Cl), for instance, gains an electron to become a chloride anion (Cl-).
Ionic Compounds and the Story of Salt
Ionic compounds are intriguing substances composed of oppositely charged ions held together by a strong electrostatic attraction. They form when atoms lose or gain electrons, resulting in ions with different charges.
Take the classic example of table salt, also known as sodium chloride. The sodium atom has one electron more than it needs, while the chlorine atom is one electron short. When these two elements come together, the sodium atom gives its extra electron to the chlorine atom, creating sodium ions (Na+) and chloride ions (Cl-).
These ions are like magnetic poles with opposite charges. The positive sodium ions are drawn to the negative chloride ions, forming an incredibly strong bond. This electrostatic attraction keeps the ions locked in a highly organized crystalline structure, where the ions line up in neat rows and columns.
The stability of ionic compounds like sodium chloride is what makes them so useful. The strong electrostatic attraction between the ions prevents them from falling apart easily. This stability is the reason salt can withstand heat, pressure, and even some chemical reactions.
Ionic compounds also have some unique properties. For instance, they tend to be hard and brittle because the ions are tightly packed and resist movement. Additionally, many ionic compounds, including salt, are soluble in water because the polar water molecules can separate the ions.
Coulomb’s Law and Its Influence on Ionic Attractions
In the realm of chemistry, the phenomenon of ionic bonding plays a pivotal role in shaping the stability and properties of numerous substances. A fundamental aspect of ionic bond formation is the electrostatic attraction between ions, which is governed by the principles of Coulomb’s law.
Coulomb’s Law: A Measure of Electrostatic Forces
Coulomb’s law provides a quantitative framework for understanding the strength of electrostatic attraction between charged particles. It states that the force of attraction or repulsion between two point charges is directly proportional to the product of their charges and inversely proportional to the square of the distance between them.
Mathematically, Coulomb’s law is expressed as:
F = k * q₁ * q₂ / r²
where:
- F is the force of attraction or repulsion
- k is Coulomb’s constant (8.9876 × 10⁹ N⋅m²/C²)
- q₁ and q₂ are the charges of the two particles
- r is the distance between the particles
Implications for Ionic Attractions
In the context of ionic bonding, Coulomb’s law sheds light on several crucial aspects:
- Charge Effects: The strength of the electrostatic attraction between ions is directly proportional to the magnitude of their charges. Higher charged ions experience stronger interactions.
- Distance Effects: The force of attraction decreases rapidly as the distance between ions increases. This means that ions must be in close proximity to generate significant electrostatic attractions.
- Anion-Cation Distances: In ionic crystals, anions and cations arrange themselves in specific patterns to maximize electrostatic attractions and minimize repulsions. These arrangements result in the formation of crystalline structures with well-defined lattice energies.
By understanding the fundamental principles of Coulomb’s law, chemists can gain valuable insights into the behavior and properties of ionic compounds, paving the way for advancements in various fields of science and technology.
Lattice Energy and the Stability of Ionic Crystals
In the realm of chemistry, ionic bonding plays a crucial role in shaping the properties of crystals. These crystals exhibit remarkable stability, and at its core lies a fundamental concept known as lattice energy.
Lattice energy is the energy required to separate all the ions in an ionic crystal into individual gas-phase ions. It serves as a measure of the strength of the electrostatic forces that hold the ions together. The greater the lattice energy, the stronger the attraction between the ions and the more stable the crystal.
Several factors influence lattice energy, including:
- Ion charges: Ions with higher charges experience stronger electrostatic attraction, leading to a higher lattice energy.
- Ion radii: Smaller ions pack more closely together, resulting in a higher lattice energy due to increased Coulombic interactions.
- Crystal structure: The arrangement of ions in a crystal lattice can affect the lattice energy. For example, crystals with a symmetrical structure generally have higher lattice energy.
Lattice energy has a profound impact on crystal stability. Crystals with high lattice energy are more difficult to break apart, making them harder and more resistant to decomposition. Conversely, crystals with low lattice energy are more likely to dissolve or decompose.
Furthermore, lattice energy influences the solubility of ionic compounds. Compounds with high lattice energy are less soluble in polar solvents because it requires more energy to separate the ions. In contrast, compounds with low lattice energy tend to be more soluble.
Understanding lattice energy is essential for comprehending the stability and properties of ionic crystals. By exploring the factors that influence lattice energy, chemists can gain insights into the behavior and applications of these important materials.
Crystalline Solids and Ionic Structure: The Building Blocks of Ionic Compounds
Ionic crystals, the solid forms of ionic compounds, possess a highly ordered three-dimensional structure that is the foundation of their unique properties. These crystals are composed of positively charged ions (cations) and negatively charged ions (anions) arranged in alternating, regular patterns. The strong electrostatic attractions between these ions hold the crystal lattice together, giving ionic solids their characteristic hardness and brittleness.
The strength of the electrostatic interactions between ions is a key factor in determining the stability and properties of ionic crystals. The higher the charge of the ions involved, the stronger the attraction and the more stable the crystal. Additionally, the smaller the ions, the closer they can approach each other, resulting in stronger electrostatic bonds.
The hardness of ionic crystals is attributed to the difficulty in breaking apart the tightly held ions within the crystal lattice. The brittleness of these solids, on the other hand, arises from the lack of flexibility in the ionic structure. When stress is applied to an ionic crystal, the ions are unable to shift or deform, leading to cleavage and fracture.
The highly organized and rigid structure of ionic crystals significantly influences their physical properties. For example, the high melting points of ionic compounds reflect the strong interionic forces that must be overcome to melt the crystal. Additionally, ionic crystals are poor conductors of electricity in the solid state due to the fixed positions of the ions within the lattice. However, when dissolved in water or other polar solvents, ionic compounds dissociate into their constituent ions, enabling them to conduct electricity through the solution.
Solubility and Conductivity of Ionic Compounds
Ionic compounds are formed through strong electrostatic attractions between positively charged ions, known as cations, and negatively charged ions, known as anions. These ionic bonds create highly stable crystalline solids that exhibit unique properties, including solubility and conductivity.
Solubility in Polar Solvents
Ionic crystals exhibit a tendency to dissolve in polar solvents, such as water. Polar solvents possess an unequal distribution of charge, with one end being slightly positive and the other slightly negative. This polarity enables polar solvents to interact with and solvate the ions in the crystal lattice.
During solvation, the positive end of the solvent molecule aligns with the negative ions (anions), while the negative end aligns with the positive ions (cations). This electrostatic attraction between the solvent molecules and the ions weakens the ionic bonds, facilitating the separation and dissolution of the ions into the solvent.
Electrical Conductivity in Aqueous Solutions
Ionic compounds are also electrolytes, meaning they dissociate into their constituent ions when dissolved in aqueous solutions. Water molecules, being polar, surround and solvate the ions, separating them from each other.
The separated ions are free to move within the aqueous solution, carrying an electrical charge. This ionic dissociation allows ionic compounds to conduct electricity. When an electrical current is applied, the positive ions (cations) migrate towards the negative electrode, while the negative ions (anions) migrate towards the positive electrode, completing the circuit and enabling the flow of electrical current.
The dissociation of ionic compounds in aqueous solutions is a crucial property that underlies many biological and industrial processes, such as nerve impulses and battery operation.