Ionic Bonding: A Comprehensive Guide To Ion Formation, Bond Strength, And Compound Stability
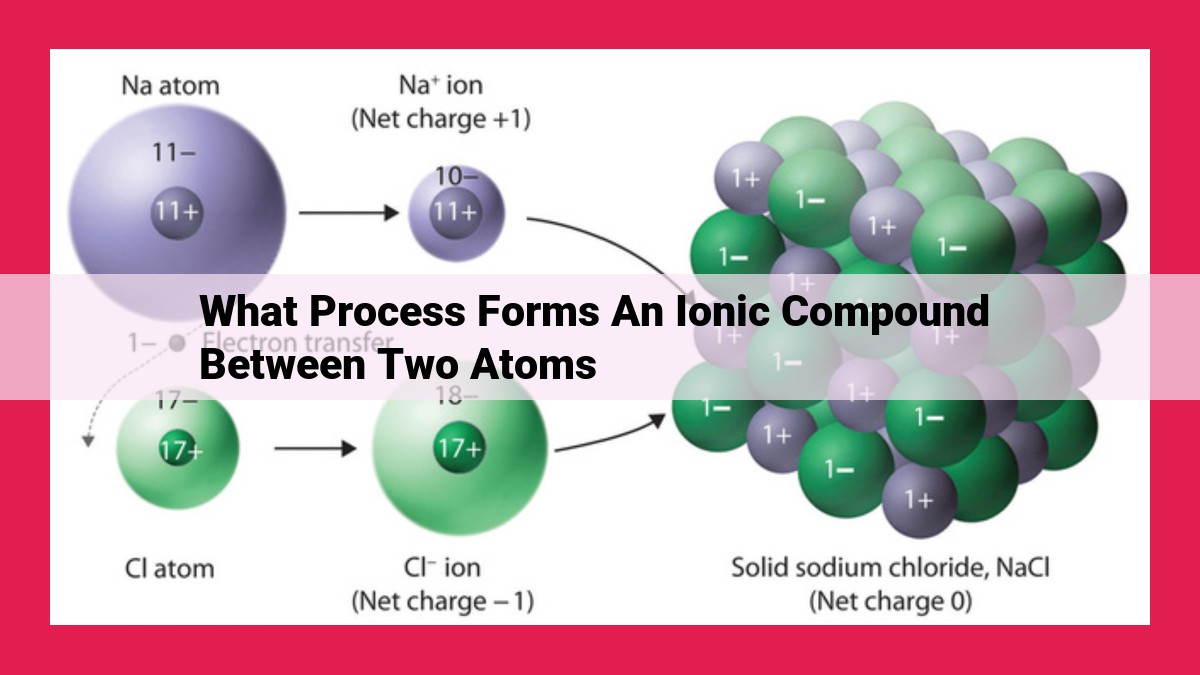
Ionic bond formation involves electron transfer between two atoms, creating positively charged cations and negatively charged anions. These ions are attracted to each other via electrostatic forces, forming an ionic compound. The ionization energy of the metal atom and the electron affinity of the non-metal atom influence the electron transfer process. The difference in electronegativity between the atoms determines the extent of ionic character in the bond, with a large difference indicating a more ionic bond. The arrangement of ions in the crystal structure influences the lattice energy, which contributes to the overall stability of the ionic compound.
Electron Transfer: The Exchange of Electrons
- Discuss how electrons flow to form ions, including oxidation and reduction reactions.
Electron Transfer: The Dance of Ions
In the fascinating world of chemistry, the electron plays a pivotal role in shaping the behavior of atoms and molecules. Electrons, with their negative charge, are like tiny dancers, constantly flowing between atoms to form ions – charged particles that drive many chemical reactions.
The Exchange of Electrons
Electron transfer is a fundamental process in chemistry, where electrons jump from one atom or molecule to another. This can happen when atoms collide or when there is an imbalance of electrons between them. The loss or gain of electrons results in ions that carry a net electrical charge.
Molecules that lose electrons become positively charged cations, while those that gain electrons become negatively charged anions. These ions can attract each other through electrostatic forces, forming ionic bonds.
Oxidation and Reduction Reactions
Electron transfer often accompanies two types of reactions: oxidation and reduction. Oxidation is the loss of electrons, while reduction is the gain of electrons. These reactions accompany each other and are always happening simultaneously.
For example, in the rusting of iron, iron atoms lose electrons (oxidation) to form positively charged iron ions. These ions then combine with oxygen molecules to form iron oxide, the familiar reddish-brown rust.
Electrostatic Attraction: The Dance of Ions
In the realm of chemistry, ions play a pivotal role, engaging in an intricate dance governed by the principles of electrostatics. Coulomb’s Law, a cornerstone of this dance, dictates the attraction between oppositely charged ions. Imagine tiny particles, like positively charged sodium ions (Na+) and negatively charged chlorine ions (Cl-), drawn together by an invisible force. The greater the charge of the ions, the stronger the attraction.
Beyond the mere attraction between ions, electrostatics also influences the polarity of molecules. Polarity refers to the uneven distribution of charge within a molecule, creating a dipole moment. In an ionic bond, the positive and negative ions are separated, resulting in a permanent dipole moment. This dipole moment plays a crucial role in determining the properties and behaviors of ionic compounds.
In ionic bonding, the attraction between oppositely charged ions is the driving force that holds the compound together. The strength of this attraction, known as lattice energy, depends on several factors, including the charges of the ions and the arrangement of ions within the crystal lattice. Lattice energy is a measure of the energy required to separate the ions in a crystal.
The electronegativity of an atom, its ability to attract electrons, also influences the polarity of an ionic bond. In any given bond, the atom with the higher electronegativity will have a greater share of the electrons, resulting in a larger dipole moment and a stronger ionic bond.
Understanding electrostatics and its role in ionic bonding is essential for comprehending the diverse array of properties exhibited by ionic compounds. From their high melting and boiling points to their ability to conduct electricity, the dance of ions governed by electrostatic principles underlies the behavior of these fascinating materials.
Ionization Energy: The Release of Electrons
Imagine atoms as tiny, electrified worlds where electrons whirl around the nucleus like a celestial dance. Ionization energy is the energy required to pluck one of these electrons away from this atomic ballet, creating a positively charged ion.
This energetic feat is crucial in the formation of cations, atoms that lose an electron and gain a positive charge. The lower the ionization energy, the more easily an element can shed electrons to become cations. Metals, with their loosely bound outer electrons, have a lower ionization energy compared to non-metals. This explains why metals willingly donate electrons to form positive ions.
On the other hand, non-metals have a higher ionization energy, indicating their reluctance to part with their electrons. Their tightly bound electrons demand a greater energy input to break free from the atom’s grasp. Consequently, non-metals tend to accept electrons to form negative ions.
In summary, ionization energy is the gateway to creating positively charged cations. Metals, with their low ionization energies, are well-suited for this role, while non-metals, with their higher ionization energies, prefer the company of electrons to form anions.
Electron Affinity: The Attraction for Electrons
In the captivating dance of chemical bonding, electron affinity plays a crucial role in the formation of anions, negatively charged ions. Electron affinity measures the tendency of an atom to attract and hold on to an extra electron. It’s like a cosmic tug-of-war between atoms and electrons, with some atoms winning the battle more easily than others.
As we navigate the periodic table, we discover that non-metals have a stronger appetite for electrons, boasting higher electron affinities than their metallic counterparts. This preference stems from the arrangement of their electrons. Non-metals have more empty valence orbitals, those outermost electron shells, waiting to welcome an additional electron. Metals, on the other hand, have their valence shells filled or nearly filled, making them less eager to acquire more electrons.
The strength of electron affinity directly influences the ability of an atom to form anions. The higher the electron affinity, the more easily an atom can gain an electron and become an anion. This difference in electron affinity between non-metals and metals drives the formation of ionic bonds, the chemical bonds formed between positive and negative ions.
Lattice Energy: The Adhesive Force Binding Ions
In the captivating realm of ionic bonding, understanding the forces that hold positively charged cations and negatively charged anions together is crucial. Enter *lattice energy*, a pivotal concept that governs the stability and strength of ionic compounds.
Defining Lattice Energy
Lattice energy, symbolized by “*U*“, represents the energy required to separate all the ions in a crystal lattice into an infinite number of gaseous ions. This energy is released when ions come together to form the crystal.
Impact on Ionic Bond Strength
The magnitude of lattice energy dictates the strength of an ionic bond. A *higher lattice energy* implies a *stronger bond*, as it takes more energy to break apart the ions. Conversely, *lower lattice energy* indicates a *weaker bond*.
Crystal Structure and Lattice Energy
The arrangement of ions within a crystal lattice significantly influences lattice energy. A tightly packed lattice structure leads to *higher lattice energy*, due to the increased electrostatic attraction between the ions. Conversely, a loosely packed lattice results in *lower lattice energy*.
Lattice energy is a fundamental property of ionic compounds that plays a pivotal role in determining their stability and bond strength. Understanding lattice energy provides insights into the nature of ionic bonding and the behavior of ionic compounds in various chemical systems.
Electronegativity Difference: The Measure of Ionic Character
In the realm of chemistry, understanding the nature of chemical bonds is crucial. Among various bond types, ionic bonds stand out as the electrostatic attraction between oppositely charged ions. The electronegativity difference plays a pivotal role in determining the degree of ionic character in a bond.
Defining Electronegativity
Electronegativity is a measure of an atom’s ability to attract electrons towards itself. It quantifies the tendency of an atom to form anions, negatively charged ions. The greater the electronegativity of an atom, the more strongly it attracts electrons.
Electronegativity Difference and Bond Type
The electronegativity difference between two atoms involved in a chemical bond determines the type of bond formed. When the difference is small, the bond will be covalent, characterized by the sharing of electrons between the atoms. However, when there is a significant electronegativity difference, the more electronegative atom will attract electrons away from the less electronegative atom, resulting in the formation of ions.
Ionic Character
Ionic character describes the extent to which a bond exhibits the properties of ionic bonds. These properties include the electrostatic attraction between ions and the formation of a crystal lattice. A large electronegativity difference leads to a higher degree of ionic character in the bond.
The greater the difference in electronegativity, the more the electrons are pulled towards the more electronegative atom. This creates a polarization in the bond, where the more electronegative atom becomes partially negative and the less electronegative atom becomes partially positive. As the polarization increases, the ionic character of the bond strengthens.
In summary, the electronegativity difference between two atoms is a crucial factor in determining the type of chemical bond formed. A large electronegativity difference results in a higher degree of ionic character, characterized by the formation of ions and electrostatic attraction between the oppositely charged species.