Ionic Bonding: Electrostatic Attraction, Lattice Energy, And Stability
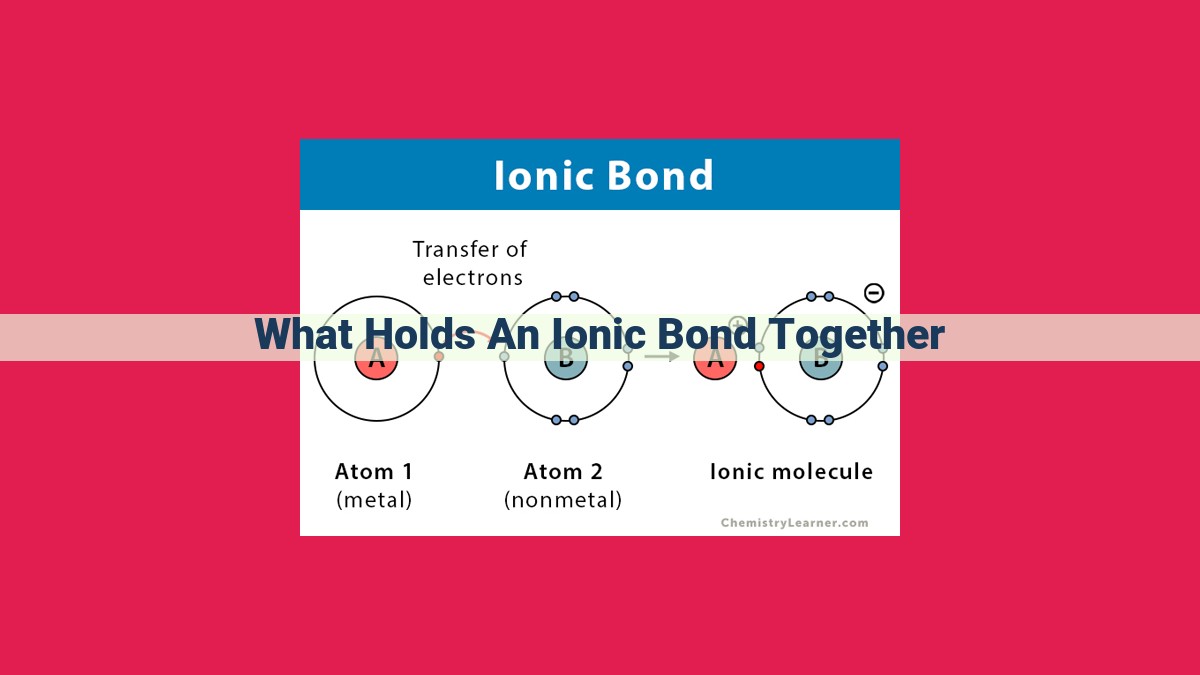
Ionic bonds are held together by the electrostatic attraction between oppositely charged ions. Coulomb’s law quantifies this force, which is proportional to the product of the charges and inversely proportional to the square of the distance between them. The lattice energy, which reflects the total attractive force within an ionic crystal, is determined by electrostatic attraction. Ionization energy, the energy required to remove an electron from an atom, and electron affinity, the energy released when an electron is added to an atom, influence the electrostatic attraction in ionic bonds. Furthermore, hydration energy, released when ions interact with polar water molecules, stabilizes ionic compounds in aqueous environments.
Electrostatic Attraction: The Force that Binds Ions Together
Ionic bonds are the glue that holds together many of the essential components of our world, from the salt we sprinkle on our food to the concrete that forms our roads. These bonds are formed through a fascinating interplay of electrostatic attraction, the force that governs the interactions between charged particles.
Electrostatic attraction is the force that exists between two oppositely charged particles. In the case of ionic bonds, the charged particles are ions, atoms or molecules that have gained or lost electrons, giving them a net electrical charge. When ions of opposite charges are brought together, they experience a strong electrostatic attraction that pulls them together.
The strength of the electrostatic attraction between ions is determined by several factors, including the size and charge of the ions. The larger the ions, the greater the distance between their nuclei, resulting in a weaker attraction. Similarly, the greater the charge of the ions, the stronger the attraction.
Coulomb’s Law: Unraveling the Force of Attraction in Ionic Bonds
In the realm of chemistry, the formation of ionic bonds is governed by the fundamental principle of electrostatic attraction. This force plays a crucial role in the stability and properties of ionic compounds. One of the key concepts in understanding electrostatic attraction is Coulomb’s law.
Coulomb’s Law: A Guiding Force
Coulomb’s law is a mathematical formula that describes the electrostatic force between two charged particles. It states that the force is directly proportional to the product of the charges and inversely proportional to the square of the distance between them. Mathematically, it can be expressed as:
Force = (k * q1 * q2) / r^2
Where:
- k is the Coulomb constant (8.9876 x 10^9 N·m²/C²)
- q1 and q2 are the charges of the particles
- r is the distance between the particles
Calculating Electrostatic Force: Uniting Charges and Distance
Using Coulomb’s law, we can calculate the electrostatic force between two ions. For instance, in sodium chloride (NaCl), the sodium ion has a charge of +1 and the chloride ion has a charge of -1. The distance between the ions is approximately 0.282 nm. Plugging these values into the formula, we get:
Force = (8.9876 x 10^9 N·m²/C²) * (1 * 1.602 x 10^-19 C) * (-1 * 1.602 x 10^-19 C) / (0.282 x 10^-9 m)^2
Force = 8.2 x 10^-9 N
This value represents the electrostatic attraction between a sodium ion and a chloride ion in NaCl.
Examples of Electrostatic Interactions in Ionic Compounds
Electrostatic attraction is not limited to the simple NaCl example. It manifests itself in various ionic compounds:
- In potassium fluoride (KF), the potassium ion (+1) and fluoride ion (-1) experience a force of 4.7 x 10^-9 N.
- In magnesium oxide (MgO), the magnesium ion (+2) and oxide ion (-2) have a stronger attraction of 26.6 x 10^-9 N.
Lattice Energy: The Strength of Ionic Bonds
In the realm of chemistry, where atoms dance and molecules form, ionic bonds are a crucial force that holds compounds together. One key concept that governs the strength of these bonds is lattice energy.
Unraveling Lattice Energy
Lattice energy, denoted as U, measures the energy required to separate all the ions in a crystal lattice into gaseous ions. It’s calculated using the formula:
U = -E * (N⁺ * N⁻) / r
where:
- E is the elementary charge (1.602 × 10⁻¹⁹ coulombs)
- N⁺ is the number of positive ions
- N⁻ is the number of negative ions
- r is the distance between the ions
The Electrostatic Dance
The relationship between electrostatic attraction and lattice energy is a tale of two opposites. Electrostatic attraction, the force that draws oppositely charged ions towards each other, is the driving force behind ionic bond formation. The stronger this attraction, the greater the lattice energy.
The Born-Haber Cycle: A Window into Ionic Bond Formation
The Born-Haber cycle is a graphical representation that unravels the energy changes involved in ionic bond formation. It’s a valuable tool for understanding the factors that influence lattice energy. By analyzing the enthalpy changes during the various steps of the cycle, chemists can gain insights into the stability and bonding characteristics of ionic compounds.
Lattice energy, a cornerstone of ionic bond theory, provides a quantitative measure of the electrostatic attraction between ions in a crystal lattice. By understanding lattice energy and its relationship with electrostatic attraction, scientists can gain a deeper appreciation for the forces that shape and stabilize ionic compounds, paving the way for advancements in materials science, energy storage, and beyond.
Ionization Energy: The Gateway to Ionic Bond Formation
In the realm of ionic bonding, where opposite charges dance, the concept of ionization energy emerges as a crucial player. This energy, denoted by the symbol IE, represents the energy required to remove an electron from a gaseous atom or ion. Understanding its intricate relationship with electrostatic attraction is essential for unraveling the secrets of ionic bond formation.
Electrostatic attraction, the invisible force that draws oppositely charged particles together, is the driving force behind ionic bonding. The more opposite the charges, the stronger the attraction, and the stronger the ionic bond.
Now, let’s explore how ionization energy influences electrostatic attraction. As we remove electron after electron from an atom, its positive charge increases. This increase in positive charge enhances the electrostatic attraction between the resulting cation (positively charged ion) and the anion (negatively charged ion) that forms the ionic bond.
The relationship between ionization energy and electrostatic attraction is evident in the Born-Haber cycle, a graphical representation of the energy changes involved in ionic bond formation. In this cycle, a high ionization energy corresponds to a stronger electrostatic attraction, leading to the formation of a more stable ionic compound.
In summary, ionization energy plays a pivotal role in ionic bond formation by influencing the strength of electrostatic attraction between ions. The higher the ionization energy, the stronger the attraction, and the more stable the ionic bond. This intricate relationship underscores the fundamental principles of ionic bonding and provides a deep understanding of the forces that shape the world around us.
Electron Affinity: The Enigmatic Force that Shapes Ionic Bonds
In the realm of chemistry, where atoms dance in intricate formations, electrostatic attraction plays a pivotal role in shaping the identity and properties of matter. Among the fundamental principles that govern these electrostatic interactions, electron affinity stands as a crucial force, influencing the stability and behavior of ionic compounds.
Unveiling Electron Affinity: The Quintessence of Atomic Embrace
Electron affinity, in its essence, quantifies the eagerness of an atom to embrace an electron. It represents the energy released when an isolated gaseous atom accepts an electron, forming a negatively charged ion. This avidity for electrons varies dramatically across elements and depends on factors such as atomic size, nuclear charge, and electronic configuration.
Electron Affinity’s Impact on Electrostatic Attraction: A Tale of Opposing Forces
Electron affinity plays a profound role in shaping the electrostatic attraction between ions. As an atom gains an electron, its negative charge intensifies, creating a stronger electrostatic attraction towards positively charged ions. This enhanced attraction strengthens the ionic bond formed between these oppositely charged particles.
The Interplay of Electron Affinity and Ionic Compound Stability: A Delicate Equilibrium
Electron affinity plays a critical role in determining the stability of ionic compounds. Elements with high electron affinities tend to form more stable ionic compounds because they hold onto their electrons more tightly. This stronger electrostatic attraction between the ions leads to a greater energy requirement to break the bond, ensuring the compound’s stability.
Examples of Electron Affinity in Action: Unveiling the Molecular Tapestry
In the realm of ionic compounds, electron affinity is a pervasive force that influences the behavior and properties of countless substances. For instance, the high electron affinity of chlorine contributes to the stability of sodium chloride (NaCl), a ubiquitous salt widely used in everyday applications. Similarly, the electron affinity of oxygen plays a crucial role in the formation of oxides, a diverse group of compounds found in everything from rust to building materials.
Electron affinity, the enigmatic force that governs an atom’s affinity for electrons, plays a crucial role in the formation, stability, and behavior of ionic compounds. By understanding the intricacies of electron affinity, chemists gain invaluable insights into the molecular tapestry that shapes the world around us.
Hydration Energy: The Electrostatic Dance of Ions and Water
In the realm of chemistry, ionic compounds are fascinating entities held together by an invisible force – electrostatic attraction. These ions, charged particles, embark on a captivating dance with water molecules, giving rise to a phenomenon known as hydration energy.
Hydration energy, simply put, is the energy released when ions dissolve in water. This energy stems from the electrostatic attraction between the charged ions and the polar water molecules. Water molecules, with their partial positive and negative charges, surround the ions, forming a hydration sphere.
The Strong Embrace of Hydration
This electrostatic embrace between ions and water molecules is no trivial matter. The strength of the hydration sphere depends on the charge and size of the ions. Smaller ions with higher charges experience stronger hydration, while larger ions with lower charges have weaker hydration.
Solubility and Stability: A Balancing Act
Hydration energy plays a critical role in determining the solubility and stability of ionic compounds. Ionic compounds with higher hydration energy tend to be more soluble in water. This is because the energy released during hydration overcomes the energy required to break apart the crystal lattice.
Stability and the Role of Hydration Energy
In addition to solubility, hydration energy also influences the stability of ionic compounds. Ions with strong hydration are less likely to undergo chemical reactions, as the energy required to break the hydration sphere is significant. This enhanced stability makes hydrated ions less reactive.
Hydration energy is a fundamental concept in understanding the behavior of ionic compounds in water. It is an electrostatic dance that governs solubility, stability, and the reactivity of these compounds. By delving into the complexities of hydration energy, we can appreciate the intricate forces that shape the world of chemistry and the delicate balance that exists in nature.