Thermodynamic Violation: Diagram Depicts Impossible Heat Engine
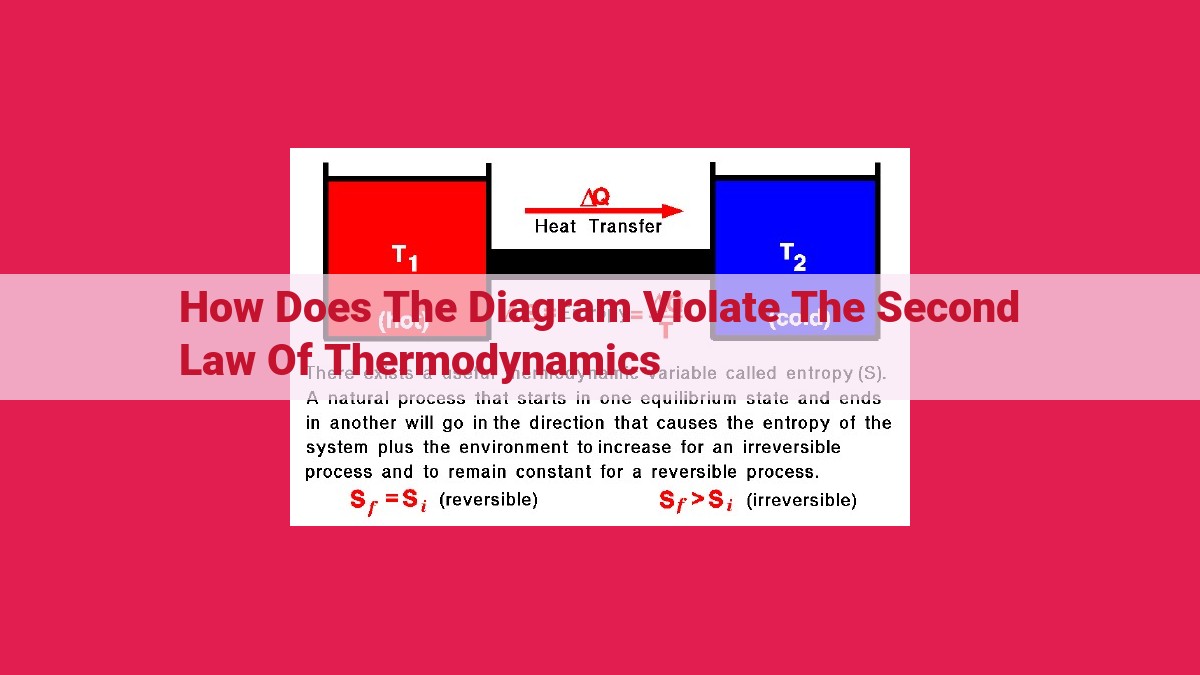
The diagram violates the Second Law of Thermodynamics, which states that entropy (a measure of disorder) in a closed system always increases. The diagram depicts a heat engine operating without a heat sink, which is impossible according to the law. Without a heat sink, the engine’s entropy would decrease, violating Clausius’s statement that heat cannot spontaneously flow from a colder to a hotter body. Additionally, the diagram lacks irreversible processes, which are essential for entropy to increase.
The Enigmatic Second Law of Thermodynamics: Unraveling the Universe’s Unstoppable Flow
In the vast tapestry of the cosmos, there exist immutable laws that govern the ebb and flow of energy, shaping the very fabric of our universe. Among these, the Second Law of Thermodynamics stands as a pillar of scientific understanding, revealing the profound influence of entropy, an elusive force that drives the inexorable march towards disorder.
This enigmatic law is more than a scientific principle; it’s a narrative that plays out in every corner of our world, from the smallest subatomic interactions to the grand cosmic dance of stars and galaxies. It’s a tale of irreversibility, where broken eggs can never be unscrambled, and heat always seeks its thermal equilibrium, like a persistent traveler yearning for a tranquil destination.
Key Concepts of the Second Law: Decoding the Language of Entropy
The heart of the Second Law lies in the enigmatic concept of entropy. Picture entropy as a measure of molecular randomness, a quantification of the chaotic dance of subatomic particles. As closed systems evolve over time, they tend towards a state of higher entropy, where order yields to disorder, like a vibrant painting slowly fading into a dull canvas.
This inexorable increase in entropy underpins three fundamental statements of the Second Law, each a distinct facet of its multifaceted nature:
Clausius’s Statement: The entropy of an isolated system not in equilibrium will tend to increase over time. In other words, the universe has a proclivity for chaos, like a pendulum forever swinging towards a state of greater disorder.
Kelvin’s Statement: It is impossible to construct a heat engine that, operating in a cycle, absorbs energy from a reservoir and does an equivalent amount of work without rejecting some of the energy to a colder reservoir. This statement highlights the inherent inefficiency of energy conversion, a reminder that the universe always takes its cut, like a mischievous imp demanding its share.
Carnot Cycle: The efficiency of a heat engine operating between two reservoirs of different temperatures is limited by the temperature difference between the reservoirs. This principle defines the theoretical limit of energy conversion efficiency, a constant reminder of the universe’s unyielding laws.
Key Concepts of the Second Law of Thermodynamics
Entropy:
Entropy measures the amount of disorder in a system. According to Clausius’s statement of the Second Law, the total entropy of an isolated system always increases over time. This means that closed systems tend to become increasingly disordered and less efficient.
Clausius Statement:
Clausius’s statement declares that heat cannot flow spontaneously from a colder object to a hotter object. This principle explains why we observe heat flowing from hot objects to cold objects, not the reverse. In real-world scenarios, this law helps us understand the direction of heat flow in systems like heat engines and refrigerators.
Kelvin Statement:
Kelvin’s statement of the Second Law states that it is impossible to create a heat engine with 100% efficiency. This means that even the best-designed heat engines will always lose some energy as heat. Kelvin’s statement has implications for the efficiency of power plants, engines, and other heat-dependent technologies.
Carnot Cycle:
The Carnot cycle is a theoretical cycle that represents the most efficient possible heat engine. It involves four processes: isothermal expansion, adiabatic expansion, isothermal compression, and adiabatic compression. Irreversible processes within the cycle, such as friction and heat loss, reduce the efficiency of real-world heat engines compared to the Carnot cycle.
Diagram Analysis:
- Identify the processes depicted in the given diagram.
- Explain how these processes violate either Clausius’s or Kelvin’s statement.
- Discuss the potential irreversibilities present in the diagram.
Diagram Analysis: Unveiling the Secrets of Entropy
Imagine a meticulously crafted diagram, a snapshot of physical processes seemingly defying the laws of nature. As we delve into this enigmatic illustration, we become detectives seeking to unravel the mysteries of the Second Law of Thermodynamics.
Identifying the Suspects:
The diagram presents a series of interconnected processes, each with its own unique characteristics. Through careful observation, we discern the presence of heat transfer, energy transformations, and the elusive entropy. These processes hold the key to understanding the law’s intricate workings.
Violation of Clausius’s Statement:
Upon closer inspection, we notice a process that transfers heat from a colder object to a hotter object. This brazen act directly contradicts Clausius’s statement, which proclaims that heat can only flow spontaneously from a hotter to a colder reservoir. The diagram’s defiance of this fundamental principle signals the presence of an irreversible process.
Kelvin’s Statement Under Fire:
Another process within the diagram depicts energy conversion. Energy flows from a higher-grade form, such as electrical energy, to a lower-grade form, such as thermal energy. This transformation runs afoul of Kelvin’s statement, which asserts that heat cannot be entirely converted into useful work in a cycle. The irreversible nature of this process further supports the violation of the Second Law.
Exposing the Irreversibility:
The diagram’s processes reveal irreversibilities that contribute to an increase in entropy. Friction, for example, dissipates energy as heat, creating disorder and raising entropy. Heat conduction through a temperature gradient also contributes to entropy’s relentless march.
The intricate dance of processes within the diagram unveils the profound implications of violating the Second Law. Irreversibilities abound, driving entropy ever higher. The diagram serves as a cautionary tale, reminding us of the fundamental principles that govern the universe.
Remember, the Second Law of Thermodynamics is an unwavering guardian of the natural order, ensuring that chaos and disorder triumph over the fleeting allure of perfection.
Irreversibility and The Inescapable Embrace of Entropy
In the realm of thermodynamics, the Second Law stands as an unwavering sentinel, guarding the boundaries of physical transformations. It whispers a tale of entropy, a subtle force that governs the irreversible dance of the universe.
Irreversible Processes: The Road Less Traveled
Irreversibility paints a stark contrast to the familiar world of reversibility. Reversible processes, like the steady swinging of a pendulum, can be reversed, leaving no trace of their existence. However, irreversible processes, such as the shattering of a glass, leave an imprint on the tapestry of the universe.
Entropy’s Shadowed Hand
Entropy, the enigmatic force that accompanies irreversibility, is defined as the measure of disorder or uncertainty in a system. Like a creeping mist, it seeps into processes, gradually eroding the order and coherence once present. Irreversible processes, with their relentless march forward, invariably lead to an increase in entropy.
Common Irreversibilities and Their Entropy-Boosting Ways
Everyday life is a playground of irreversible processes, each contributing to the inexorable rise of entropy. Diffusion, the mingling of gases or liquids, and viscosity, the resistance to fluid flow, are common examples. In these processes, organized molecular movements give way to a less ordered, more entropic state.
The irreversible processes depicted in the diagram serve as poignant illustrations. The irreversible heat transfer from a hot reservoir to a cold reservoir exemplifies the relentless flow of thermal energy, always seeking equilibrium. The mixing of gases showcases the inexorable movement towards a more disordered, homogenous state.
Entropy’s Triumph: A Universe Destined for Disorder
The universe, as we know it, is locked in an eternal battle against entropy’s relentless advance. While local pockets of order may emerge, such as the intricate structures of living organisms, the overall trend is towards increasing entropy.
This fundamental law governs everything from the decay of radioactive elements to the inevitable heat death of the universe. Entropy, like an unseen puppeteer, shapes the very fabric of our existence, reminding us of the ephemeral nature of order and the irreversible march of time.
Implications of Violating the Second Law: A Profound Departure from Reality
Imagine a world where perpetual motion machines, devices that generate endless energy without any input, are commonplace. The mere thought of such a machine challenges the very foundations of physics, specifically the Second Law of Thermodynamics. This unwavering law governs the behavior of energy and entropy in our universe. If its principles were to be violated, our comprehension of the physical world would crumble.
Perpetual Motion Machines: A Paradoxical Fantasy
Perpetual motion machines are alluring concepts that have captivated inventors and dreamers alike. The idea of a device that generates energy out of thin air is seductive. However, the Second Law of Thermodynamics stands as an insurmountable barrier to such machines. It states that the total entropy of an isolated system can only increase or remain constant, never decrease. In other words, disorder always increases, making the creation of a perpetual motion machine impossible.
Heat Engine Efficiency: Bounded by Thermodynamic Limits
Heat engines are devices that convert heat into mechanical energy. The efficiency of these engines is determined by the temperature difference between the heat source and the heat sink. According to the Second Law of Thermodynamics, the efficiency of any heat engine cannot exceed the Carnot efficiency, which is determined by the temperatures of the heat source and sink. This fundamental limit ensures that no heat engine can perfectly convert heat into work, thus further emphasizing the significance of the Second Law.
By understanding the implications of violating the Second Law of Thermodynamics, we gain a deeper appreciation for the order and balance that govern our universe. The law serves as a constant reminder that perpetual motion is a fantasy and that heat engines have inherent limitations. These principles guide our technological advancements and shape our understanding of the physical world.