Structure And Components Of Hemoglobin: Understanding The Oxygen-Binding Protein
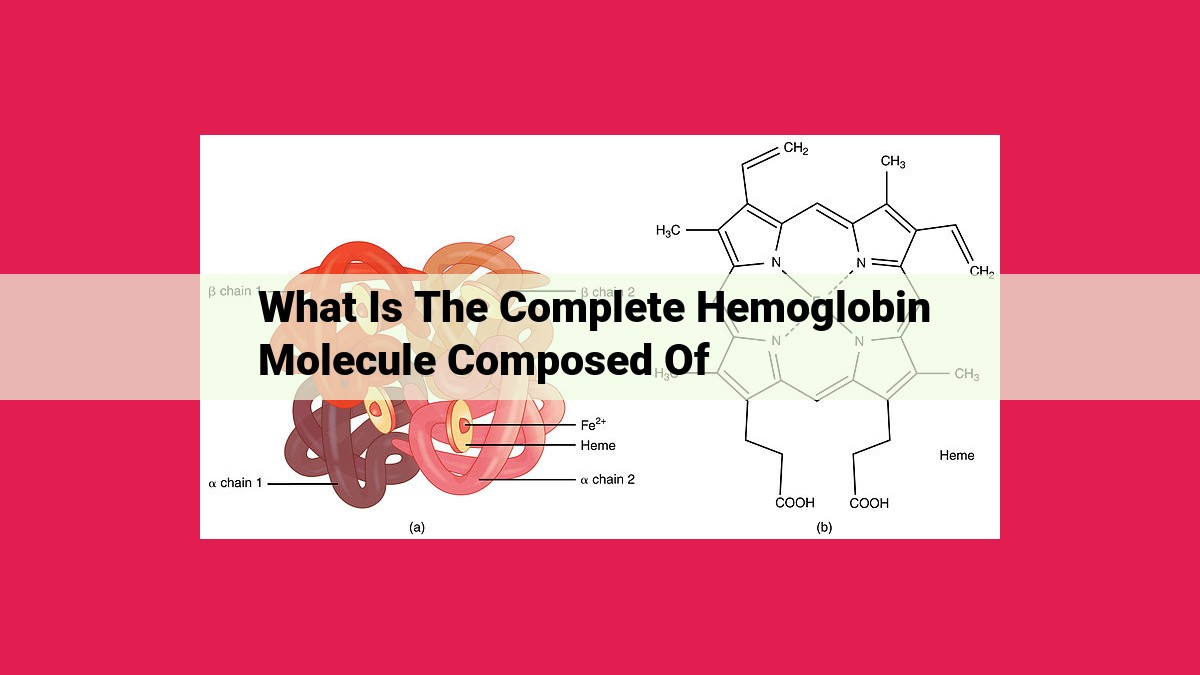
The complete hemoglobin molecule is composed of four components: 1) a porphyrin ring structure with an iron ion and axial ligands, known as heme, which binds oxygen; 2) globin, a protein matrix of polypeptide chains arranged in a tetrameric structure; 3) alpha and beta chains, two types of globin subunits with similar structures and functions; 4) heme pockets, hydrophobic regions in the globin matrix where oxygen binds and is released.
Heme: The Oxygen-Binding Cofactor
In the intricate dance of life, oxygen plays a pivotal role, fueling our every breath and sustaining our metabolic engines. At the heart of this oxygen-binding ballet lies a heme molecule, a complex and mesmerizing cofactor.
Heme’s foundation is a porphyrin ring, a flat, aromatic structure adorned with 24 carbon atoms and 12 nitrogen atoms. Nestled at the center of this ring is an iron ion (Fe2+), the essential conductor of oxygen’s embrace.
To ensure the stability of this complex, two axial ligands extend from the iron ion: one from the histidine residue of the surrounding protein (globin) and the other from oxygen itself. This delicate arrangement allows heme to reversibly bind oxygen, a critical step in the transportation and utilization of life’s vital gas.
Globin: The Protein Matrix
Introduction:
Hemoglobin, the oxygen-carrying protein in our red blood cells, doesn’t work alone. It relies on a crucial component called globin. Globin serves as the protein matrix, providing the structural framework that houses and protects the oxygen-binding elements, heme groups.
Polypeptide Chains and Quaternary Structure:
Globin is a complex protein composed of multiple polypeptide chains. These chains fold and interact in a specific way to create the three-dimensional quaternary structure of hemoglobin. The most common form of hemoglobin, found in adult humans, is a tetramer, consisting of two alpha and two beta polypeptide chains.
Globin Fold and Heme Pockets:
The polypeptide chains in globin fold into a characteristic globin fold. This intricate structure features deep heme pockets, which are hydrophobic environments that accommodate the heme groups. The heme groups are nestled within the globin fold, held in place by various amino acid residues. This strategic arrangement ensures the stability and function of hemoglobin.
Conclusion:
Globin, the protein matrix, plays a vital role in hemoglobin’s function. Its polypeptide chains and quaternary structure create a stable scaffold, while its precise folding forms heme pockets that provide a secure environment for oxygen binding. Without globin, hemoglobin would be unable to effectively transport oxygen throughout the body, making life as we know it impossible.
Quaternary Structure: The Hemoglobin Assembly
Hemoglobin stands tall among life’s molecular marvels, its purpose as grand as its structure. This intricate protein molecule comprises four globin subunits, each tightly bound in a tetrameric embrace to create a nearly spherical protein complex. Their union is not merely a matter of proximity but a precisely orchestrated dance, held together by a symphony of non-covalent interactions.
The globin subunits, like celestial bodies orbiting a star, arrange themselves in two pairs: alpha-alpha and beta-beta. These pairs form dimers, which then intertwine to create the final tetrameric structure. Like a master jigsaw puzzle, each subunit fits seamlessly into its designated position, forming a cooperatively binding ensemble that governs the essential task of oxygen transport.
The non-covalent bonds that orchestrate this molecular masterpiece contribute to its stability and flexibility. Hydrogen bonds, like tiny molecular bridges, connect the subunits, while hydrophobic interactions ensure that the protein’s interior remains shielded from the watery environment. These interactions, like the threads of a delicate tapestry, weave together the hemoglobin assembly, enabling it to withstand the rigors of its life-sustaining mission.
The cooperative nature of hemoglobin’s oxygen binding is a crucial adaptation. As one subunit binds to oxygen, it triggers a conformational change that enhances the binding affinity of the remaining subunits for this vital gas. This allows hemoglobin to efficiently load up on oxygen in the lungs and release it where it’s needed most – the tissues and cells that fuel our daily existence.
In essence, hemoglobin’s quaternary structure is a testament to the exquisite precision of nature’s designs. Its tetrameric assembly, stabilized by non-covalent interactions, forms the foundation for the cooperative oxygen binding that defines its critical role in life’s symphony.
Hemoglobin Subunits: Alpha and Beta Chains
At the heart of hemoglobin’s critical role in oxygen transport lies the intricate interplay between its two types of globin subunits: alpha and beta chains. These polypeptides form the protein matrix that houses the oxygen-binding heme cofactors. While each chain possesses unique characteristics, together they paint a harmonious picture of structural and functional synergy.
Amino Acid Composition and Differences
Each alpha and beta chain comprises a unique sequence of amino acids, giving rise to their distinct molecular fingerprints. Alpha chains encompass 141 amino acid building blocks, while beta chains extend slightly longer with 146 amino acids. These differences in length contribute to subtle variations in their overall shape and properties.
Structural and Functional Similarities
Despite their variations, alpha and beta chains share a remarkable structural similarity known as the “globin fold.” This common architecture features eight helical segments arranged in a specific configuration. These helices create a hydrophobic core that sequesters the heme molecules essential for oxygen binding.
Nestled within these helical folds lies a crucial structural element: the heme pocket. Comprising a highly conserved set of amino acid residues, these pockets provide a tailored environment for heme binding and facilitate the reversible uptake and release of oxygen.
Cooperativity and the Symphony of Subunits
The remarkable feature of hemoglobin stems from the cooperative interaction between its alpha and beta subunits. This intricate interplay enables the molecule to regulate its oxygen-binding affinity based on the surrounding oxygen concentration.
When oxygen levels are low, alpha and beta subunits undergo conformational changes that increase their affinity for the gas. Conversely, as oxygen abundance rises, these subunits shift their conformation, reducing their affinity and promoting oxygen release.
Summary
The alpha and beta subunits of hemoglobin are distinct yet complementary entities that orchestrate a harmonious symphony of structural and functional properties. Their unique amino acid composition, shared globin fold, and coordinated movements underpin the molecule’s ability to bind, transport, and release oxygen with unmatched efficiency. Together, these subunits form the foundation of hemoglobin’s critical role in sustaining life.
Heme Pockets: The Binding Sites for Oxygen
Within the depths of our red blood cells, a remarkable duo orchestrates oxygen delivery throughout our bodies: hemoglobin and its crucial cofactor, heme. At the heart of this partnership lies the heme pocket, a molecular sanctuary where oxygen finds its temporary home.
The heme pocket is a hydrophobic haven, a sanctuary shielded from water by a cozy embrace of amino acid residues. These residues, like protective guardians, form a barrier that excludes water while inviting oxygen’s embrace. Among them, histidine stands out as a key player, its imidazole ring reaching out to bind to the iron ion at the center of heme, securing oxygen’s grip.
How does oxygen find its way into this hidden chamber? The secret lies in the conformational changes that hemoglobin undergoes. When oxygen is absent, the heme pocket adopts a “tense” state, with the iron ion pulled closer to the histidine residue. This conformational shift creates a higher affinity for oxygen, drawing it into the pocket like a magnet.
As oxygen enters, the heme pocket undergoes a subtle transformation, transitioning into a “relaxed” state. The iron ion retreats, allowing oxygen to bind more tightly. This shift in conformation triggers a cascade of events, altering the interactions between the hemoglobin subunits and ultimately facilitating the cooperative binding of multiple oxygen molecules.
Once bound within the heme pocket, oxygen is shielded from premature release. The hydrophobic environment and the tight embrace of the amino acid residues ensure that oxygen remains captive until it reaches its intended destination. And when tissues demand oxygen, the heme pocket undergoes another conformational shift, releasing oxygen into the bloodstream to meet the body’s needs.
Thus, the heme pocket serves as a sophisticated docking station for oxygen, orchestrating its binding, storage, and release with remarkable precision. Without this molecular marvel, the vital process of oxygen delivery would falter, leaving our cells starved of the life-sustaining gas they crave.