Glycolysis: Exploring The Final Products Under Aerobic And Anaerobic Conditions
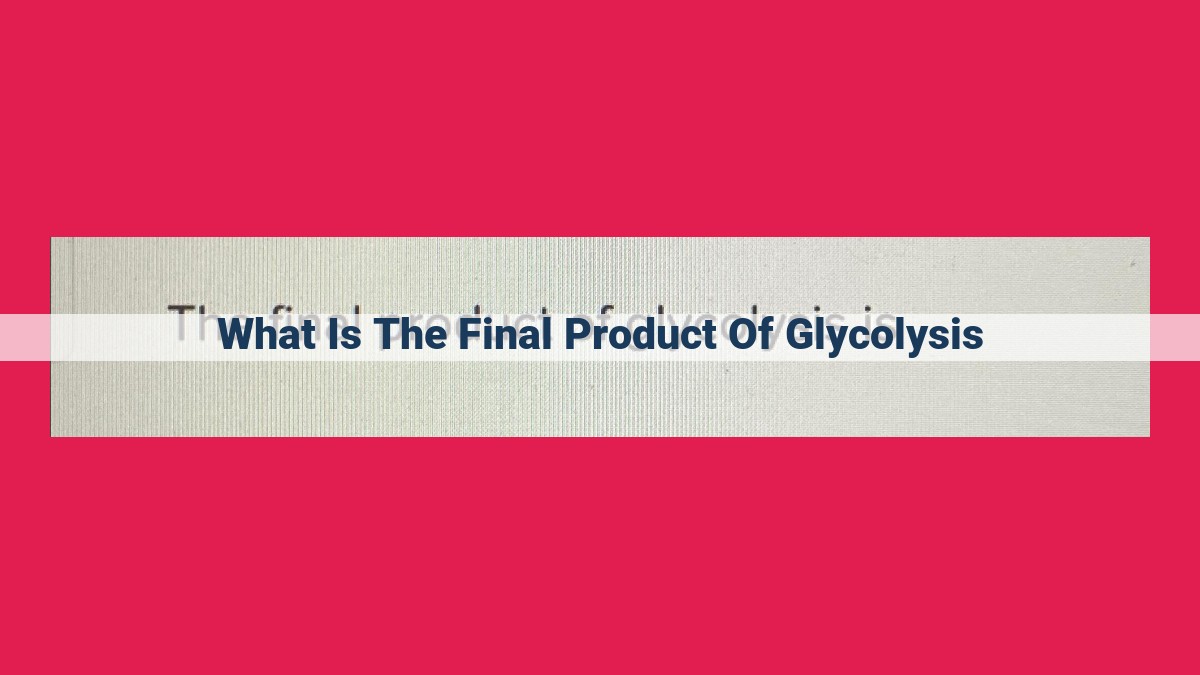
Final Product of Glycolysis
Under aerobic conditions, the final product of glycolysis is pyruvate, a three-carbon molecule. However, under anaerobic conditions, such as during strenuous exercise, pyruvate is converted to lactate through the process of fermentation. This conversion regenerates NAD+, which is essential for glycolysis to continue, allowing the production of additional energy in the absence of oxygen.
Embark on the Journey of Glycolysis: Unraveling the Secrets of Cellular Energy Production
In the realm of life, energy reigns supreme, and glycolysis stands as a cornerstone of this energetic dance. This intricate metabolic pathway orchestrates the breakdown of glucose, the lifeblood of our cells. So, let’s take a plunge into the fascinating world of glycolysis and uncover its profound significance in sustaining life.
Defining Glycolysis: The Energy-Generating Powerhouse
Glycolysis is a biochemical symphony consisting of a series of intricate reactions that transform glucose into energy-rich molecules. This process serves as the foundation for energy production in virtually all living organisms. It’s like a cellular power plant, converting the potential energy stored in glucose into usable forms that fuel our bodies.
The Enigmatic Process of Glycolysis: Unraveling the Ten Enzymatic Reactions
Introduction:
Glycolysis, the cornerstone of cellular metabolism, is an intricate dance of enzymatic reactions that transforms glucose, our body’s primary fuel source, into pyruvate. This fundamental process underpins energy production in virtually every cell and paves the way for subsequent metabolic pathways.
Stage I: Glucose Activation and Phosphorylation
The journey begins with glucose phosphorylation, catalyzed by hexokinase, followed by glucose-6-phosphate isomerase, which converts glucose-6-phosphate to its fructose equivalent.
Stage II: Cleavage and Conversion to Glyceraldehyde-3-Phosphate
The six-carbon fructose-6-phosphate is cleaved by phosphofructokinase-1 into two three-carbon molecules, dihydroxyacetone phosphate (DHAP) and glyceraldehyde-3-phosphate (G3P). Triose phosphate isomerase ensures equilibrium between DHAP and G3P.
Stage III: Substrate-Level Phosphorylation and Energy Harvest
G3P, the central metabolite of glycolysis, embarks on a series of reactions that result in the formation of 1,3-bisphosphoglycerate. This process, catalyzed by glyceraldehyde-3-phosphate dehydrogenase and phosphoglycerate kinase, yields two molecules of ATP, the cellular currency of energy.
Stage IV: Rearrangements and Electron Transfer
Phosphoglycerate isomerase rearranges 1,3-bisphosphoglycerate to 3-phosphoglycerate, which is subsequently dehydrated by phosphoglycerate mutase to produce 2-phosphoglycerate. Enolase catalyzes the removal of water, generating phosphoenolpyruvate (PEP), a high-energy molecule. Finally, pyruvate kinase transfers a phosphate group from PEP to ADP, yielding a second molecule of ATP and the final product of glycolysis, pyruvate.
Conclusion:
The ten enzymatic reactions of glycolysis orchestrate a meticulous transformation of glucose into pyruvate, releasing valuable energy in the form of ATP. This process serves as the foundation for cellular metabolism, powering essential functions and providing a gateway to further energy-generating pathways.
The Final Destination of Glycolysis: Aerobic vs. Anaerobic Twist
In the realm of cellular metabolism, glycolysis stands as a crucial pathway, unlocking the energy stored within glucose molecules. It’s a fascinating tale of enzymatic reactions, substrate transformations, and the dance between aerobic and anaerobic conditions.
Under the spotlight of aerobic conditions, where oxygen is readily available, glycolysis culminates in the production of pyruvate, a three-carbon molecule. This pyruvate embarks on a journey to the mighty Krebs cycle, where it undergoes further energy-yielding transformations.
However, when anaerobic conditions prevail and oxygen becomes scarce, glycolysis takes a different turn. Pyruvate, instead of venturing into the Krebs cycle, undergoes a clever transformation to lactate. This switch is primarily driven by the need to regenerate NAD+, an essential electron carrier that keeps the glycolysis engine running.
The conversion of pyruvate to lactate by lactate dehydrogenase is a pivotal step in anaerobic metabolism. This enzymatic dance ensures a continuous supply of NAD+ to support glycolysis and maintain cellular functions amidst oxygen deprivation.
In a nutshell, glycolysis’s final product hinges on the availability of oxygen. Aerobic conditions lead to pyruvate production, while anaerobic conditions trigger a shift towards lactate formation to replenish NAD+. This metabolic flexibility allows cells to adapt to varying oxygen levels and maintain energy production, even in challenging environments.
Importance and Applications of Glycolysis
Glycolysis is a fundamental metabolic pathway that underpins cellular energy metabolism. It plays a pivotal role in converting glucose, the primary fuel source for cells, into energy-rich molecules.
Glycolysis generates two molecules of pyruvate during cellular respiration. This pyruvate then enters the Krebs cycle, another critical metabolic pathway. Within the Krebs cycle, pyruvate undergoes further reactions to release additional energy and generate more adenosine triphosphate (ATP), the cellular energy currency.
Moreover, glycolysis is also a precursor to oxidative phosphorylation, the final stage of cellular respiration that produces the vast majority of ATP in the cell. By funneling glucose-derived pyruvate into these subsequent metabolic pathways, glycolysis ensures a continuous supply of energy to power cellular processes.
Additional Considerations for Glycolysis
Physiological Significance of Glycolysis
Glycolysis is not just an isolated pathway; it’s a crucial player in the body’s energy metabolism. The products of glycolysis, such as pyruvate and ATP, are essential for subsequent metabolic processes like the Krebs cycle and oxidative phosphorylation. These pathways work in conjunction to generate high-energy molecules that fuel various cellular activities.
Clinical Implications of Glycolysis Dysregulation
Understanding glycolysis is crucial not only for basic biology but also for its clinical implications. Dysregulation of glycolysis can lead to various diseases. For instance, in certain types of cancer, glycolysis is abnormally increased, known as the Warburg effect. This elevated glycolysis provides cancer cells with the energy they need for rapid proliferation.
Role in Red Blood Cells
Glycolysis is of particular importance in red blood cells, which lack mitochondria. Red blood cells rely solely on glycolysis for energy production. This unique reliance on glycolysis highlights its critical role in maintaining the oxygen-carrying capacity of red blood cells.
Historical Background
The discovery and understanding of glycolysis have a rich history. The initial framework of the pathway was established in the early 20th century by biochemists like Gustav Embden and Otto Meyerhof. Their groundbreaking work paved the way for subsequent research that further elucidated the complexities of glycolysis.
Applying Glycolysis to Daily Life
The principles of glycolysis can be found in our daily lives. For example, fermentation is a process that utilizes glycolysis to convert sugars into ethanol in beverages like beer and wine. This biochemical transformation exemplifies the practical applications of glycolysis beyond the confines of a laboratory setting.