Glycolysis: Unlocking Cellular Energy Through Glucose Breakdown
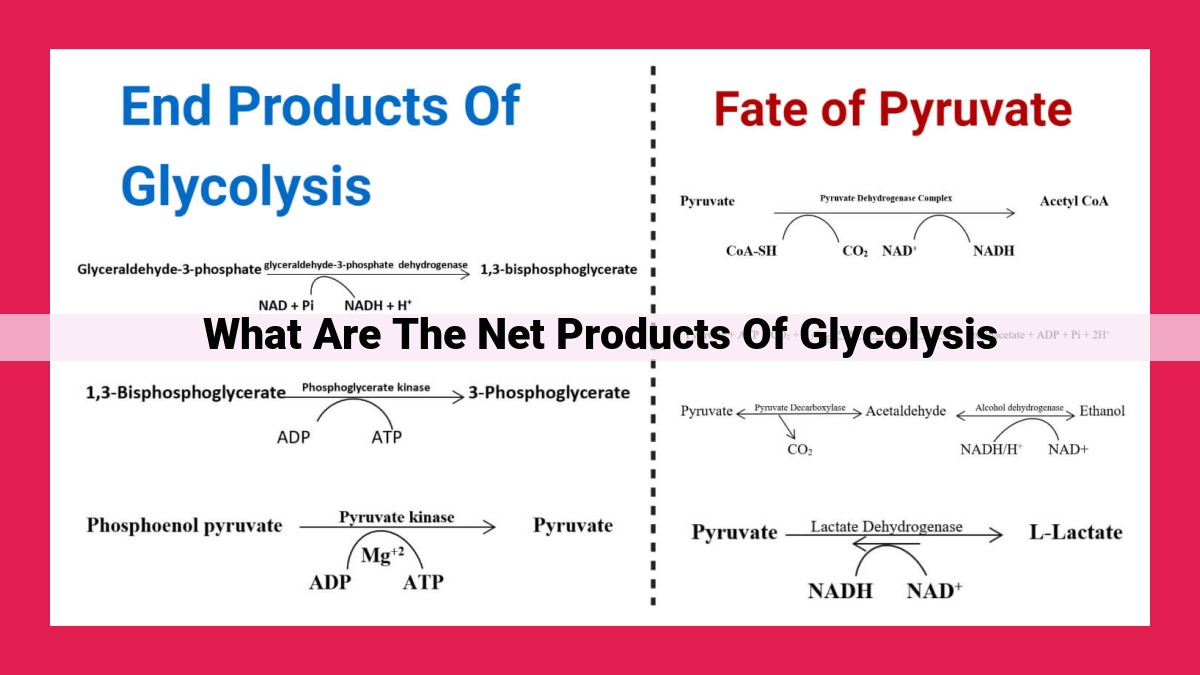
Glycolysis, the first step of cellular respiration, breaks down glucose into pyruvate, the primary net product. Additionally, glycolysis generates ATP, the cellular energy currency, and NADH, a high-energy electron carrier. These products play vital roles in cellular energy metabolism. Pyruvate can be further metabolized to produce ATP, while NADH donates electrons to the electron transport chain, leading to the production of significant amounts of ATP.
Glycolysis: The Initiation of Energy Production
In the realm of cellular life, energy is the driving force that fuels every process. At the heart of this energy production lies glycolysis, a pivotal metabolic pathway that ignites the journey of converting glucose into usable energy.
Overview of Glycolysis
Glycolysis is the initial stage of both cellular respiration and fermentation, two essential processes for extracting energy from glucose. This pathway takes place in the cell’s cytoplasm and serves as the starting point for breaking down glucose, the primary source of energy for most living organisms.
The Role of Glycolysis in Breaking Down Glucose
During glycolysis, a molecule of glucose is decomposed into two molecules of pyruvate. This process involves a series of enzymatic reactions that extract energy by phosphorylating glucose and cleaving it into smaller molecules. As glucose is broken down, electrons are captured by NAD+ (nicotinamide adenine dinucleotide), creating high-energy NADH molecules. These NADH molecules will later be used in oxidative phosphorylation to generate ATP, the cellular energy currency.
Pyruvate: The Primary Net Product of Glycolysis
Within the intricate tapestry of cellular respiration, there exists a pivotal molecule known as pyruvate. It emerges as the primary net product of glycolysis, the initial stage of this energy-generating pathway. Pyruvate holds immense significance in cellular energy metabolism and beyond.
Production of Pyruvate: The Culmination of Glycolysis
Glycolysis, a series of complex chemical reactions, breaks down glucose, the body’s primary energy source. Through a series of enzymatic transformations, glucose is gradually broken down, yielding two molecules of pyruvate. This process is akin to dismantling a complex structure, with pyruvate representing the final, fundamental building blocks.
Significance of Pyruvate in Energy Metabolism
Pyruvate serves as a crucial intermediate in the broader energy-yielding pathways of the cell. It can either be converted into acetyl-CoA and enter the Krebs cycle for further energy production or undergo fermentation to generate lactic acid or ethanol under anaerobic conditions. This versatility enables cells to adapt their energy production to varying environmental conditions.
Role in Gluconeogenesis: The Reverse Pathway
Beyond its energetic significance, pyruvate also plays a role in gluconeogenesis, the process of synthesizing glucose from non-carbohydrate sources. Under conditions of glucose scarcity, the body can convert pyruvate back into glucose through a series of reactions, ensuring a constant supply of this vital molecule for essential cellular functions.
In conclusion, pyruvate stands as a pivotal molecule in cellular energy metabolism. As the primary net product of glycolysis, it serves as a crucial intermediate in both catabolic and anabolic pathways. Its significance extends beyond energy production, reaching into the realms of gluconeogenesis and cellular adaptation. Understanding the role of pyruvate is paramount in unraveling the intricate tapestry of biochemical processes that sustain life.
ATP: The Cellular Energy Currency
In the bustling realm of cellular life, energy reigns supreme, fueling every intricate process that keeps our bodies humming. Among the molecular players that make this energy magic happen, ATP stands out as the undisputed champion. This remarkable molecule serves as the cellular energy currency, powering an astonishing array of cellular activities.
Within the intricate ballet of glycolysis, the initial stage of cellular respiration, ATP takes center stage through a clever dance called substrate-level phosphorylation. Here, high-energy phosphate groups are transferred from certain molecules directly onto ADP (adenosine diphosphate), transforming it into the energized ATP.
Think of ATP as the Energizer Bunny of the cell, the ultimate source of power for an astounding array of cellular chores. From muscle contractions to nerve impulses and chemical reactions, ATP is the tireless workhorse that keeps the cellular machinery humming. Its high-energy phosphate bonds act like tiny batteries, releasing bursts of energy when the cell needs a boost.
Without ATP, the cell would be like a car without fuel, unable to perform its vital functions. It’s the driving force behind essential processes such as protein synthesis, ion transport, and the pumping of molecules against concentration gradients. In short, ATP is the lifeblood of cellular existence, the essential ingredient that fuels the symphony of life.
NADH: The Vital Electron Carrier in Cellular Energy Production
In the intricate dance of cellular respiration, NADH emerges as a crucial player, akin to a high-energy courier tasked with transporting electrons. These electrons, brimming with potential, embark on a pivotal journey that ultimately fuels the production of the cellular energy currency, ATP.
As glycolysis sets the stage for energy production by breaking down glucose, NADH stands ready to capture H+ ions and electrons, converting these components into NADH molecules. This transformation infuses NADH with substantial energy, which it then carries to the mitochondria, the cell’s energy powerhouse.
Within the mitochondria, the electron transport chain awaits, a series of protein complexes that serve as a relay for NADH and its electron cargo. As electrons are passed from complex to complex, they undergo a cascade of energy-releasing reactions. This energy is used to pump H+ ions across the mitochondrial membrane, creating a concentration gradient.
The accumulated H+ ions eagerly rush back down their electrochemical gradient through a specialized enzyme called ATP synthase. As they surge through, their movement harnesses the energy to drive the formation of ATP, the universal energy currency of cells.
The involvement of NADH in oxidative phosphorylation, the process that generates most of the ATP in our cells, underscores its vital role in sustaining cellular life. Without the efficient transport of electrons by NADH, the production of ATP would stall, and our cells would be deprived of the energy they need to function.