How Glucose Fuelizes Cells: Understanding Atp Production Through Glycolysis And Oxidative Phosphorylation
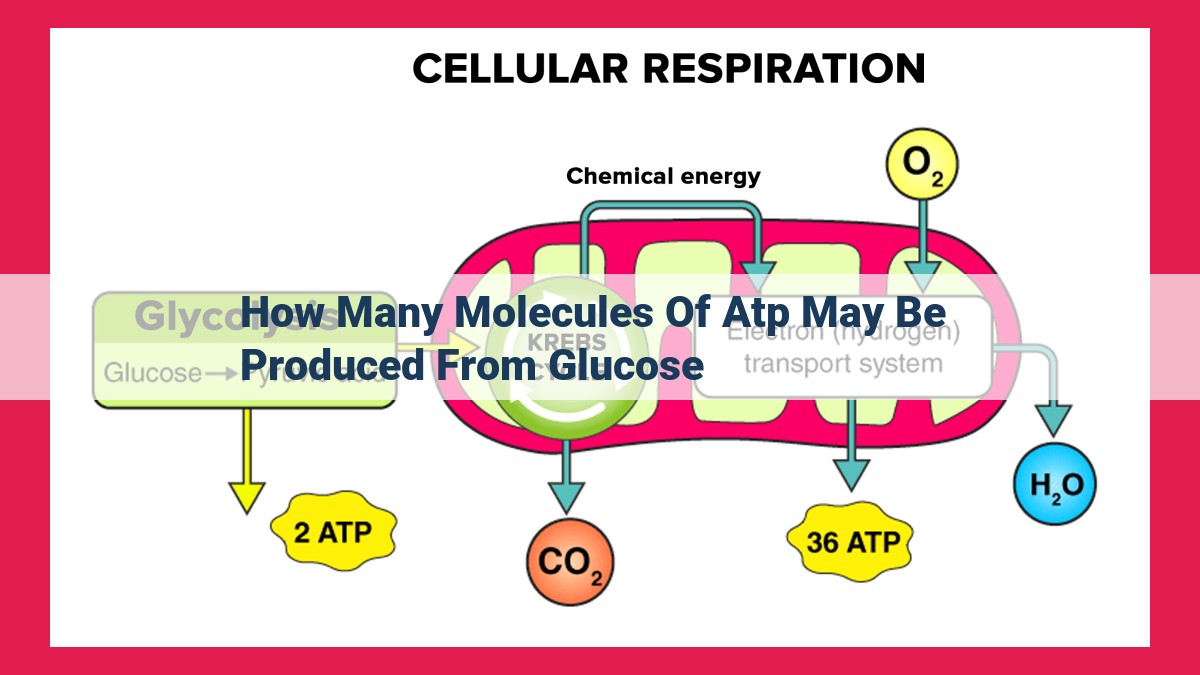
Glucose, a crucial cellular energy source, undergoes glycolysis, the TCA cycle, and oxidative phosphorylation to generate ATP. Glycolysis yields 2 ATP, while the TCA cycle and electron transport produce approximately 34-36 ATP molecules. This process results in a total of approximately 36-38 ATP molecules generated per glucose molecule, providing cells with the energy they require for various cellular processes.
- Explain the role of glucose as a vital energy source for cells.
Glucose: The Lifeline of Cellular Energy
In the bustling world of our body, cells are the microscopic powerhouses that fuel our every move. And just like any bustling city, cells need a steady supply of energy to function. Enter glucose, the star energy source for our hard-working cells.
Glucose is a simple sugar molecule that our bodies break down to release energy. Think of it as the fuel that powers our cellular engines. Without glucose, our cells would be like cars running on empty, unable to perform their vital tasks.
How Glucose Supercharges Our Cells
Glucose’s energy-releasing journey begins in a process called glycolysis. This is where glucose gets broken down into even smaller molecules, capturing a modest amount of energy in the form of two ATP molecules. ATP, or adenosine triphosphate, is the cellular currency of energy, used to power all sorts of cellular activities.
Once glycolysis does its magic, the energy train keeps rolling. The newly formed molecules enter the TCA cycle, also known as the Krebs cycle or citric acid cycle. Here, the wheels really start turning, producing a hefty supply of high-energy molecules called FADH2 and NADH.
FADH2 and NADH: The Power-Generating Duo
FADH2 and NADH are like energy-packed batteries that carry electrons. These electrons then get passed along a series of proteins called the electron transport chain, a bit like runners in a relay race.
As these electrons race through the chain, they release energy that pumps protons across a membrane, creating an electrochemical gradient. This gradient is the driving force that powers the final step of glucose metabolism: oxidative phosphorylation.
During oxidative phosphorylation, protons rush back through a channel called ATP synthase, like water flowing through a turbine. As the protons flow, they cause ATP synthase to rotate, generating even more ATP molecules.
The Energy Payoff: A Glucose Powerhouse
In total, the breakdown of one glucose molecule through glycolysis and oxidative phosphorylation yields a whopping 36-38 ATP molecules. That’s a lot of energy to keep our cells humming!
So, there you have it, the remarkable journey of glucose, the vital energy source that fuels our cellular engines. It’s a complex process, but one that’s essential for sustaining life.
Glycolysis: The Initial Energy Yield
Embark on a captivating journey into the realm of energy metabolism, where we unravel the intricacies of glucose’s transformation into a vital energy source for our cells. This odyssey begins with glycolysis, the initial phase of cellular respiration that kick-starts the process of extracting energy from glucose.
As glucose embarks on its metabolic adventure, it encounters a series of enzymes that orchestrate a cascade of chemical reactions. These reactions gradually break down glucose, a six-carbon molecule, into two molecules of pyruvate, each containing three carbons. Along this metabolic pathway, glycolysis also orchestrates the net production of two ATP molecules.
ATP: The Cellular Currency of Energy
ATP, or adenosine triphosphate, stands as the primary energy currency of cells. Its high-energy phosphate bonds serve as reservoirs of chemical energy, which cells can readily tap into to power a myriad of cellular activities, from muscle contraction to nerve impulses.
During glycolysis, the production of ATP occurs through a substrate-level phosphorylation mechanism. Here, an enzyme directly transfers a phosphate group from a high-energy intermediate to ADP (adenosine diphosphate), resulting in the synthesis of ATP.
Although glycolysis only generates a modest net gain of two ATP molecules, it serves as a crucial foundation for subsequent stages of cellular respiration, where the bulk of energy extraction takes place. Stay tuned for our next chapter in this exciting energy metabolism saga, where we delve into the TCA cycle and electron transport chain.
Acetyl-CoA: The Gateway to the TCA Cycle
In our energy-guzzling cells, glucose takes center stage as the ultimate fuel source. Through a series of intricate biochemical reactions, glucose is transformed into energy-rich molecules that power our every move and thought.
One crucial step in this energy-generating cascade is the formation of acetyl-CoA, the key to unlocking the mysteries of the TCA cycle. Let’s venture into the cellular powerhouse, the mitochondria, to witness this fascinating process.
As glucose undergoes glycolysis, it’s broken down into two molecules of pyruvate. Each pyruvate molecule undergoes further processing, losing a molecule of carbon dioxide and combining with coenzyme A to form acetyl-CoA. This high-energy molecule serves as the gateway to the tricarboxylic acid (TCA) cycle, also known as the Krebs cycle.
The TCA cycle is a circular pathway, a metabolic dance where acetyl-CoA plays a starring role. As acetyl-CoA enters the cycle, it combines with a four-carbon molecule called oxaloacetate to form a six-carbon compound called citrate. Citrate then undergoes a series of enzymatic transformations, releasing carbon dioxide, FADH2, and NADH molecules.
FADH2 and NADH are the energy-carrying molecules that will later power the electron transport chain, the final stage of cellular respiration where most ATP molecules are generated. As the TCA cycle continues, oxaloacetate is regenerated, ensuring the cycle’s continuous operation.
FADH2 and NADH Production in the TCA Cycle
As glucose journeys through the TCA cycle, it undergoes a series of enzymatic reactions that extract energy from its carbon bonds. Within this intricate dance of biochemical transformations, two key electron carriers, FADH2 and NADH, emerge as pivotal players.
FADH2 and NADH: The Powerhouse Molecules
FADH2 and NADH are electron transport molecules that accept high-energy electrons during specific reactions in the TCA cycle. In the Krebs cycle, citric acid, the starting molecule, undergoes a sequence of transformations, each liberating energy. These reactions involve the transfer of electrons to FADH2 and NADH, trapping the energy within their chemical bonds.
The Role of FADH2 and NADH in Electron Transport
The electrons captured by FADH2 and NADH embark on a critical mission in the electron transport chain (ETC). Embedded in the inner mitochondrial membrane, the ETC resembles a series of electron-pumping stations, where these molecules donate their electrons. As the electrons cascade through the ETC, energy is released, which is used to pump protons across the mitochondrial membrane.
Oxidative Phosphorylation: Energy from Proton Pumping
The proton gradient established by the ETC provides the driving force for oxidative phosphorylation, a process that produces ATP. ATP, the cellular energy currency, powers the myriad of processes within our cells. Therefore, FADH2 and NADH, by donating electrons to the ETC, indirectly contribute to the generation of ATP.
The Significance of FADH2 and NADH in Energy Metabolism
The production of FADH2 and NADH in the TCA cycle is a crucial step in the energy production pathway of glucose. These molecules facilitate the electron transfer that ultimately enables the ETC to generate ATP, the essential fuel for cellular activities. Without these electron carriers, the process of glucose oxidation would be incomplete, depriving our cells of the energy they need to thrive.
ATP Generation: The Powerhouse of Cells
In our cellular universe, glucose reigns supreme as the energy currency that fuels every living process. However, unlocking this energy requires a series of intricate steps, where glycolysis and the TCA cycle prepare glucose for its ultimate transformation.
But the real magic happens in the electron transport chain. This bustling molecular highway is where FADH2 and NADH molecules, the high-energy carriers from glycolysis and the TCA cycle, come into play. As these molecules shuttle along the chain, they donate electrons like eager hands passing a relay baton.
With each electron handover, protons are pumped across a membrane, creating an electrochemical gradient like a tiny battery. This gradient then drives the final stage of energy production: oxidative phosphorylation.
The ATP synthase, a molecular turbine, harnesses this gradient’s power to spin. As it turns, it adds inorganic phosphate to ADP, creating the energy-storing molecule ATP. It’s like riding a bicycle dynamo that cranks out ATP with every revolution.
This relentless electron flow, proton pumping, and ATP generation is a cellular masterpiece, the epitome of nature’s efficiency. It’s all thanks to the FADH2 and NADH molecules that donate their precious electrons, enabling us to move, think, and experience the wonders of life.
Glucose Oxidation: Unveiling the Energy Powerhouse Within
Imagine your cells as tiny engines, constantly buzzing with activity. And just like engines need fuel to operate, our cells rely on glucose, a vital energy source, to power their cellular machinery. Glucose provides the necessary fuel for our cells to perform their life-sustaining functions, from muscle contractions to brainpower.
Glycolysis: The First Spark of Energy
When glucose enters our cells, it undergoes a process called glycolysis, the first step in its energy-yielding journey. During glycolysis, the glucose molecule is broken down into two molecules of pyruvate, releasing a modest amount of energy in the form of 2 ATP molecules. These 2 ATP molecules represent the initial energy gain from glucose oxidation.
Acetyl-CoA and the TCA Cycle: A Deeper Dive into Energy Production
The pyruvate molecules produced in glycolysis are further broken down and converted into a molecule called acetyl-CoA. Acetyl-CoA then enters the TCA cycle, also known as the Krebs cycle, where it’s combined with oxygen to generate additional energy. As the cycle progresses, it produces more ATP, along with energy-rich molecules called FADH2 and NADH, which play a crucial role in subsequent energy production.
Electron Transport and Oxidative Phosphorylation: The Energy-Generating Duo
FADH2 and NADH, the molecules generated in the TCA cycle, act as the fuel for another energy-generating process called electron transport. They pass their electrons along an electron transport chain, releasing energy that is used to pump protons across a membrane. This proton gradient drives oxidative phosphorylation, where the majority of ATP molecules are produced.
Total ATP Yield from Glucose Oxidation: A Grand Energy Harvest
Combining the energy gains from glycolysis, the TCA cycle, and oxidative phosphorylation, we arrive at the astonishing total ATP yield from glucose oxidation. Approximately 36-38 ATP molecules are produced for every molecule of glucose that enters the cellular energy-generating machinery. This remarkable energy harvest fuels our cells and powers the myriad of life processes that sustain us.