Galvanometers: Unlocking Electrical Insights And Optimizing Electronic Systems
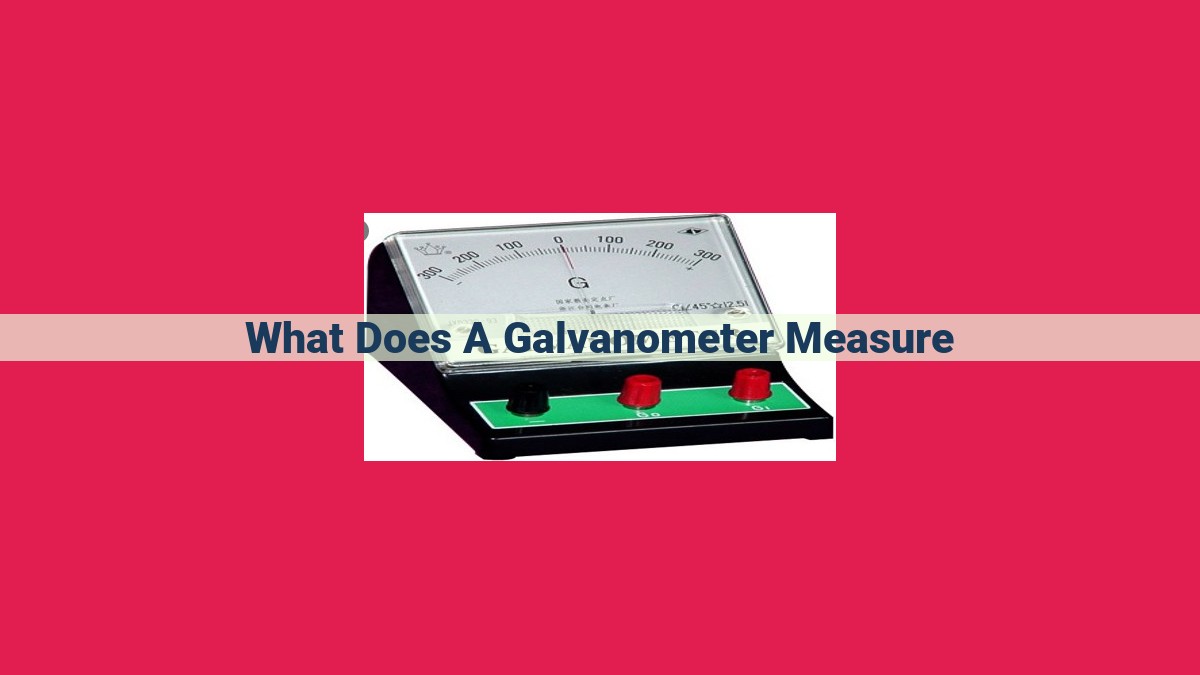
A galvanometer, an instrument that detects and measures electric current, is a crucial tool in electrical measurements. It senses the flow of charge through a circuit, providing insights into the magnitude and direction of current. Galvanometers enable the exploration of fundamental electrical concepts such as charge, voltage, resistance, and power, uncovering the relationships between these quantities. By measuring current, galvanometers facilitate the study of electrical circuits, helping engineers design and optimize electronic systems.
Electrical Measurements: Exploring the Fundamentals with a Galvanometer
In the realm of electrical engineering, understanding how electricity flows and behaves is crucial. Measuring these electrical phenomena accurately is equally important, and that’s where the galvanometer comes into play.
A galvanometer is a sensitive instrument that detects and measures the presence and direction of small electrical currents. Its origins can be traced back to the 19th century, when scientists like Luigi Galvani and Alessandro Volta were pioneering the field of electricity. The galvanometer’s purpose is to reveal the hidden world of electric charge and current flow.
The working principle of a galvanometer is based on the interaction between a current-carrying coil and a magnetic field. When an electric current passes through the coil, it creates a magnetic field that interacts with the external magnetic field, causing the coil to rotate. The amount of rotation is directly proportional to the strength of the current.
Galvanometers are used in various applications, including:
- Detecting small electrical currents
- Measuring the direction of current flow
- Calibrating other electrical instruments
- Studying the magnetic properties of materials
They are essential tools in electrical engineering laboratories, research institutions, and industries that deal with electrical systems and components.
Chapter 2: Current – The Flow of Charge
In the realm of electricity, current is the invisible force that animates our devices, enabling them to perform their countless functions. It’s the ceaseless movement of charge, the fundamental building block of electricity. Imagine a river, its waters flowing relentlessly, carrying energy from one point to another. In the electrical world, current is that river, and charge is its flowing water.
Measuring Current
Measuring current is like gauging the flow rate of a river. Scientists have devised a unit for this purpose – the ampere (A), named after André-Marie Ampère, the French physicist who made significant contributions to the understanding of electricity. A single ampere represents the flow of one coulomb of charge per second. Charge, in turn, is the electrical property of an object that determines the strength of its interactions with other charged objects.
Charge and Resistance
Charge comes in two forms: positive and negative. Positive charge is found in an excess of protons within an atom, while negative charge is found in an excess of electrons. These tiny particles are in constant motion, colliding with each other and other particles. In a conductor, such as a metal wire, these collisions allow charge to flow freely. However, some materials offer resistance to this flow, like a pebble-strewn riverbed slowing down the current. This property is known as resistance. Resistance is measured in ohms (Ω), named after Georg Ohm, who first described its mathematical relationship with current and voltage.
The dance between current, charge, and resistance is a delicate balance that governs the flow of electricity in our gadgets and devices. Understanding this fundamental aspect of electricity is crucial for unlocking the secrets of the electrical realm.
Voltage: Electrical Potential Difference
Think of voltage as the driving force that pushes current (the flow of electric charge) through a circuit. It’s like the pressure in a water pipe—the higher the pressure, the faster the water flows. In electricity, voltage is measured in volts (V).
Potential difference is the difference in voltage between two points in a circuit. Imagine a battery with positive and negative terminals. The positive terminal has a higher voltage than the negative terminal, creating a potential difference. This difference drives electrons from the negative terminal, through the circuit, and back to the positive terminal.
Electrical potential is the energy stored in an electric charge. It’s a measure of how much work the charge can do. The potential difference between two points is the difference in their electrical potential. So, voltage is the driving force that moves charges, creating a potential difference and causing current to flow.
Resistance: The Obstacle in Electrical Flow
In the realm of electricity, resistance plays a crucial role in shaping the flow of electrons. Imagine a river where water seeks the path of least resistance, flowing effortlessly through wide channels. However, if obstacles like rocks and narrow passages hinder its path, the rate of flow slows down. Similarly, in an electrical circuit, resistance acts as an impediment to the smooth flow of current.
Resistance, often symbolized by the letter R, measures the degree to which a material opposes the passage of electric charge. It is akin to friction in mechanical systems, creating a barrier that hinders the free movement of electrons. The higher the resistance, the greater the hindrance, and the less current can flow.
The relationship between resistance, current (I), and voltage (V) is expressed by Ohm’s law: V = IR. This equation elegantly illustrates that voltage, the driving force behind current flow, is directly proportional to resistance and current. As resistance increases, voltage must increase to maintain the same current. Conversely, a decrease in resistance allows for greater current flow at a given voltage.
Understanding resistance is essential for designing and analyzing electrical circuits. By carefully selecting materials and components with appropriate resistance levels, engineers can control the flow of current, ensuring the efficient and safe operation of devices from household appliances to complex electronic systems.
Power: The Energy Flow in Electrical Circuits
In the realm of electricity, power reigns supreme as the fundamental measure of energy flow. It plays a crucial role in understanding how electrical devices operate, from tiny transistors to massive generators.
Defining Power
Electrical power measures the rate at which energy is transferred or converted within a circuit. It is expressed in watts (W), a unit named after the esteemed Scottish inventor, James Watt. One watt represents the power consumed or produced when one joule of energy is transferred or converted per second.
Measuring Power
Measuring power is straightforward using a device called a wattmeter. This instrument measures both the current flowing through a circuit and the voltage across it. The product of these two measurements yields the power in watts.
Power’s Complicated Relationship with Voltage and Current
The relationship between power, voltage, and current is elegantly captured by the equation:
Power (P) = Voltage (V) x Current (I)
This formula highlights two important aspects:
-
Power is directly proportional to voltage and directly proportional to current. Increasing either voltage or current results in an increase in power.
-
Power is measured in watts (W), voltage in volts (V), and current in amperes (A).
Power, the lifeblood of electrical systems, is a measure of the energy flow within a circuit. Understanding power is critical for designing and operating electrical devices efficiently. The equation P = VI serves as a powerful tool for calculating power, enabling engineers and technicians to optimize the performance of countless electrical applications.
EMF: Electromotive Force
In the realm of electricity, there exists an unseen force that compels the flow of electrons and sparks the dance of current. This enigmatic entity is known as electromotive force, abbreviated as EMF.
Imagine a bustling metropolis, where charge carriers (electrons) are like cars traversing a vast network of roads (conductors). EMF acts as the driving force, the invisible hand that propels these cars along their paths.
EMF originates from various sources, such as batteries, generators, and even chemical reactions. It represents the energy required to move a unit of charge around a closed circuit. Just as a higher water pressure drives a stronger flow of water through a pipe, a greater EMF propels a more vigorous current through a conductor.
EMF possesses an intrinsic relationship with charge and electrical potential. Every moving charge generates a tiny electrical field, and the potential difference between two points is simply the cumulative effect of these fields. EMF, therefore, acts as the catalyst, driving charges from higher potential to lower potential, creating a continuous circuit of electrical flow.
Magnetic Field: Current-Induced Phenomena
In the realm of electricity, where electrons dance and circuits hum, an invisible force emerges: the magnetic field. This field, like a celestial tapestry, weaves its way around any conductor when current surges through it. It’s a story of intertwined phenomena, where charge, current, and the magnetic field itself engage in an intricate waltz.
The magnetic field, like a loyal bodyguard, surrounds the conductor, its strength proportional to the current’s intensity. It forms a halo of force, with every moving charge contributing its invisible thread to the magnetic fabric. This symphony of forces creates a swirling vortex around the conductor, a testament to the intertwined nature of electricity and magnetism.
But how does this magnetic field come to life? It all begins with the movement of charges. When electrons race through a wire, their motion creates a magnetic field. It’s as if each electron carries a tiny magnet, aligning with its neighbors to form a collective magnetic field that permeates the space around the conductor. The greater the current, the stronger the magnetic field, as more electrons contribute their magnetic contributions.
The magnetic field, in turn, exerts its influence on the moving charges. It exerts a force on them, causing them to experience a magnetic push or pull. This force can even cause moving charges to change their direction, as they dance to the tune of the magnetic field’s influence.
It’s a mesmerizing interplay, where current gives birth to magnetic fields, and magnetic fields shape the movement of current. Together, they form an inseparable bond, revealing the intricate tapestry of electromagnetic phenomena that governs our electrical world.
Electrical Potential: The Power of Charge Distribution
In the realm of electricity, electrical potential stands as a cornerstone concept that governs the behavior of charged particles. It represents the energy per unit charge stored within an electric field, enabling the calculation of the force exerted on charges placed within that field.
The magnitude of electrical potential at a given point is directly proportional to the net charge responsible for creating the field and inversely proportional to the distance from the source charge. Mathematically, it is expressed as:
V = k * Q / r
where:
- V represents the electrical potential
- k is Coulomb’s constant (8.98755 × 10^9 N m^2 / C^2)
- Q is the charge creating the field
- r is the distance from the source charge
The relationship between electrical potential, charge, and voltage is crucial in understanding the dynamics of electrical circuits. Voltage, a measure of potential difference, quantifies the electrical potential difference between two points in a circuit. It plays a fundamental role in driving the flow of current and transferring energy within electrical systems.
The concept of electrical potential is essential for analyzing and designing circuits, as it governs the movement of charges and the interactions between different components. By manipulating the electrical potential, engineers can control the behavior of electrical devices and optimize their performance.
Charge: The Foundation of Electricity
The very foundation of electricity lies in a fundamental entity known as charge. Everything around us, from the chair you’re sitting on to the air you’re breathing, is composed of atoms, which in turn are made up of even smaller particles called electrons and protons. These tiny particles carry charge, an intrinsic property that governs their interactions with the world around them.
Types of Charge
Charge exists in two forms: positive and negative. Electrons carry negative charge, while protons carry positive charge. The interplay between these opposite charges gives rise to the electromagnetic force that shapes our universe.
Charge and Electrical Current
Electrical current is the flow of charge through a material. When electrons move from one atom to another, they create an electrical current. The magnitude of the current depends on the number of charges flowing and the speed at which they move.
Charge and Electromotive Force (EMF)
EMF (electromotive force) is the driving force that causes charge to move. Sources of EMF, such as batteries and generators, create a potential difference between two points, which in turn drives electrons to flow.
Charge and Energy
The movement of charge through a material can result in the transfer of energy. Electrical power is the rate at which electrical energy is transferred. When electrons flow through a resistor, for instance, their energy is converted into heat.
Charge is the fundamental building block of electricity. Its interactions and movement give rise to current, voltage, and power. Understanding charge is essential for comprehending the world of electricity and the technologies it powers.