Unlocking The Significance Of The Freezing Point Of Water In Kelvin For Thermodynamics And Beyond
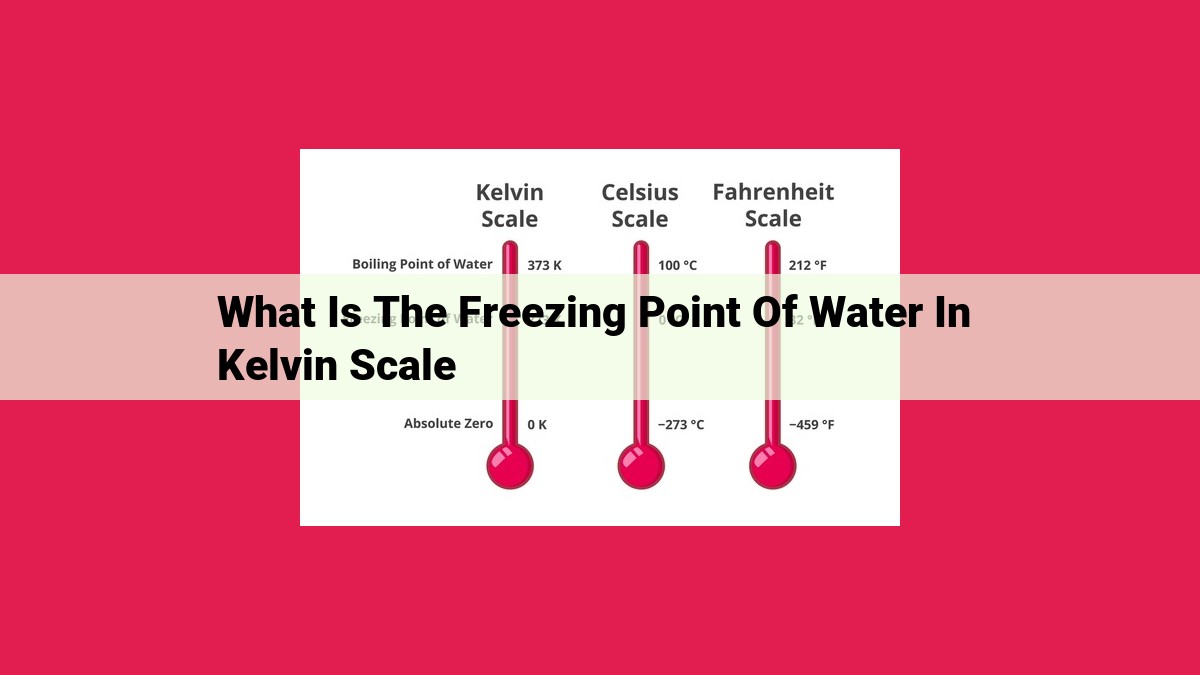
The freezing point of water, defined as the temperature at which water transforms from a liquid to a solid, is a crucial thermodynamic concept. In the Kelvin scale, the absolute temperature scale, the freezing point of water is 273.15 K. This conversion is significant because the Kelvin scale is the basis for measuring thermodynamic temperature. Understanding the freezing point of water in Kelvin deepens our comprehension of temperature, phase changes, and the properties of water, which find applications in various fields like food preservation and scientific research.
Embark on a captivating journey into the realm of thermodynamics and phase changes, where the freezing point stands as a pivotal concept. The freezing point is the temperature at which a liquid transforms into a solid, marking a crucial transition in the state of matter. Its significance extends far beyond everyday experience, providing a window into the inner workings of energy and the behavior of substances. Join us as we unravel the mysteries of the freezing point, exploring its relationship to the Kelvin scale, the Celsius scale, and the fundamental principles of phase equilibrium.
Defining the Freezing Point: A Threshold of Transformation
The freezing point is the specific temperature at which a liquid solidifies. It represents a delicate balance between the energy of the liquid molecules and the attractive forces that draw them together. As a liquid cools, its molecules lose kinetic energy, causing them to slow down. When the temperature reaches the freezing point, the molecules have lost enough energy to be overcome by the attractive forces, and they crystallize into a solid structure. This process, known as freezing, releases energy in the form of heat.
The Significance of the Freezing Point in Thermodynamics
In thermodynamics, the freezing point holds immense importance. It serves as a reference point for measuring thermodynamic properties, such as entropy, enthalpy, and Gibbs free energy. The freezing point also plays a crucial role in determining the phase behavior of substances. By studying the freezing point and other phase transitions, scientists can gain valuable insights into the molecular interactions and energy landscape of materials.
Understanding the Kelvin Scale: The Absolute Temperature Measure
In the realm of thermodynamics, temperature plays a pivotal role in understanding the behavior of matter. Among the various temperature scales we use, the Kelvin scale stands out as the absolute temperature scale, providing a fundamental reference point for scientific investigations.
The concept of absolute temperature originated from the work of Lord Kelvin, a renowned Scottish physicist. Kelvin realized that the freezing point of water could serve as a fixed reference point, but he sought a more precise and scientifically sound scale. Through his groundbreaking research, he devised the Kelvin scale, named in his honor, which is based on the concept of absolute zero.
Absolute zero is the theoretical point at which all molecular motion ceases, and no heat can be extracted from a substance. This thermodynamic zero point corresponds to -273.15°C on the Celsius scale. By adding 273.15 to the Celsius temperature, we arrive at the corresponding Kelvin temperature, establishing a direct mathematical relationship between the two scales.
For example, the freezing point of water, which is 0°C on the Celsius scale, becomes 273.15 K on the Kelvin scale. This conversion is crucial in thermodynamics and phase changes, as it allows us to express temperatures in absolute terms, providing a consistent and universal reference point for scientific calculations.
**Converting between Celsius and Kelvin: Unraveling the Absolute Scale**
Temperature, a fundamental concept in science and daily life, plays a crucial role in understanding the behavior of matter and energy. Among the countless temperature scales devised, the Celsius and Kelvin scales stand out as the most widely used in various scientific and everyday applications. While the Celsius scale is familiar to many due to its convenience in measuring the freezing and boiling points of water, the Kelvin scale holds an equally significant place in thermodynamics and physics.
The Kelvin scale, also known as the absolute temperature scale, is the foundation upon which the laws of thermodynamics are built. It is based on the concept of absolute zero, the lowest possible temperature at which all atomic and molecular motion ceases. Absolute zero is defined as -273.15°C or 0 K.
The conversion between Celsius and Kelvin scales is straightforward, making it essential for understanding various physical phenomena. The formula for converting from Celsius to Kelvin is:
Kelvin (K) = Celsius (°C) + 273.15
For instance, if the temperature is 20°C, the corresponding Kelvin temperature would be 293.15 K. Conversely, to convert from Kelvin to Celsius, the formula is:
Celsius (°C) = Kelvin (K) - 273.15
Grasping the relationship between these scales is critical, especially when dealing with the freezing point of water. By definition, the freezing point of water is 0°C or 273.15 K. Understanding this equivalence is vital for scientific research, engineering applications, and even everyday occurrences like understanding weather patterns.
Ultimately, the conversion between Celsius and Kelvin unlocks a deeper comprehension of temperature and its significance in thermodynamics. It enables scientists and engineers to analyze phase transitions, calculate energy changes, and unravel the complexities of the physical world.
The Freezing Point of Water: A Story of Temperature and Phase Change
In the realm of thermodynamics, a freezing point marks a crucial juncture, signaling a substance’s transformation from a liquid to a solid state. For water, this defining moment occurs at 0 degrees Celsius, etching itself into the annals of science and everyday life.
This pivotal point is deeply entwined with the properties of water and the interplay of temperature and phase changes. Water’s unique molecular structure endows it with an unusually high specific heat capacity. This property means that a relatively large amount of heat energy is required to raise water’s temperature by a given amount.
As we gradually cool water, its temperature decreases, reducing the intensity of molecular motion. At 0°C, a remarkable shift occurs. The molecules slow down significantly, and their ability to move past each other diminishes. This kinetic energy reduction causes water to solidify, transitioning from a liquid to a crystalline solid.
The freezing point can be visualized using a phase diagram. Here, temperature and pressure conditions are plotted, revealing the boundaries between liquid, solid, and gaseous phases. For water, the freezing point line separates the liquid and solid regions, with 0°C marking the exact boundary.
This freezing point has profound implications in our daily lives. From preserving food and making ice to cryopreserving biological samples and advancing scientific research, the freezing point of water plays an integral role. By understanding this critical temperature, we harness its power for a multitude of applications.
Temperature and the Symphony of Phase Changes
The Dance of Heat and Matter
Imagine a world where matter exists in a continuous state. However, in our reality, it’s a ballet of transformations, where temperature orchestrates the changes. As we add heat to a substance, its temperature rises, and with it, a cascade of phase changes unfolds before our eyes.
Melting: From Solid to Liquid
As you warm a solid, such as ice, its molecules gain energy, breaking the bonds that hold them rigid. The solid begins to melt, transitioning into a liquid state. This occurs at its melting point, a specific temperature characteristic of the substance. For water, this pivotal temperature is 0°C (32°F).
Boiling: Liquid’s Leap into Gas
Continuing the dance, as we heat a liquid further, its molecules become even more energetic, overcoming the cohesive forces holding them together. At its boiling point, the liquid boils, transforming into a gas. Water’s boiling point is 100°C (212°F) at sea level.
Sublimation: A Direct Path to Gas
Sometimes, a substance takes a shortcut in its phase changes. Sublimation occurs when a solid directly transitions into a gas, skipping the liquid phase entirely. This intriguing phenomenon is observed when dry ice (solid carbon dioxide) turns into a gas at room temperature.
Phase Equilibrium: A Delicate Balance
Throughout these transformations, substances reach a delicate balance known as phase equilibrium. At a specific temperature and pressure, the solid, liquid, and gas phases can coexist peacefully. The addition or removal of heat can shift this equilibrium, prompting further phase changes.
Properties of Water at Its Freezing Point
Water, a ubiquitous substance essential for life, exhibits remarkable properties when it reaches its freezing point of 0°C (32°F). These unique characteristics play a crucial role in various natural phenomena and technological applications.
At the freezing point, water’s density becomes anomalous. Unlike most substances that become denser when they solidify, water expands, resulting in a less dense solid form. This unusual behavior is attributed to the formation of hydrogen bonds between water molecules, creating a crystalline structure with open spaces. This expanded solid form is commonly observed as ice floating on liquid water.
Another fascinating property of water at its freezing point is its viscosity. Viscosity measures a fluid’s resistance to flow. Surprisingly, water’s viscosity increases as it approaches the freezing point. This increased resistance to flow contributes to the slushy consistency often observed in partially frozen water.
Finally, water’s specific heat, which measures the amount of heat required to raise the temperature of a substance, exhibits a significant change at the freezing point. Water’s specific heat _decreases as it approaches freezing. This property means that less energy is required to raise the temperature of water near its freezing point, making it more susceptible to temperature fluctuations.
Applications of the Freezing Point of Water: A Journey from Food Preservation to Scientific Advancements
The freezing point of water, a seemingly simple concept, holds a treasure trove of practical applications that impact our daily lives and push the boundaries of scientific research. Its significance extends far beyond the realm of thermodynamics and phase changes.
Food Preservation: A Timeless Technique
The freezing point of water plays a crucial role in food preservation. By lowering the temperature of food below its freezing point, microbial growth is inhibited, extending the shelf life of perishable goods. This technique has been used for centuries to ensure a safe and nutritious food supply.
Ice Making: A Summertime Essential
From refreshing beverages to icy treats, the freezing point of water forms the foundation of ice making. By freezing water at or below 0°C, we create the perfect solid to cool us down on hot days.
Cryopreservation: Preserving Life’s Potential
The freezing point of water also holds tremendous significance in cryopreservation, a process that preserves biological samples at ultra-low temperatures. By maintaining cells, tissues, and organs at or below their freezing point, scientists can halt biological processes and protect them for future use in medical research and treatment.
Scientific Research: A Window into Fundamental Phenomena
Beyond its practical applications, the freezing point of water serves as a valuable tool in scientific research. By studying how water behaves at its freezing point, scientists can gain insights into the properties of matter, phase transitions, and the fundamental laws of thermodynamics. This knowledge has led to advancements in fields such as materials science, chemistry, and biophysics.
The freezing point of water, once a simple concept confined to textbooks, has evolved into a multifaceted phenomenon with far-reaching applications. It plays a vital role in preserving food, creating icy treats, safeguarding biological samples, and fueling scientific discoveries. Understanding the freezing point of water not only enhances our understanding of thermodynamics but also reveals its profound impact on our daily lives and the progress of scientific knowledge.
Measuring the Enigma of Freezing Point
To unravel the mysteries surrounding the freezing point, scientists utilize an array of techniques, each shedding light on this pivotal aspect of thermodynamics. Among these methods, three stand out: thermometry, calorimetry, and cryoscopy.
Thermometry: Capturing the Chill
Thermometry, the art of measuring temperature, plays a central role in determining the freezing point. Using calibrated thermometers, scientists immerse the specimen in a controlled environment, gradually cooling it. As the temperature drops, the thermometer’s readings track the progress towards the freezing point. When the readings plateau, indicating a halt in the temperature drop, the freezing point has been reached.
Calorimetry: Quantifying the Heat of Fusion
Calorimetry delves deeper into the thermodynamics of freezing by measuring the heat released or absorbed during the phase transition. As water transforms from a liquid to a solid, it releases a specific amount of heat known as the enthalpy of fusion. By immersing the specimen in a calorimeter filled with a known mass of water, scientists can calculate the heat released by the specimen. This data can then be used to determine the freezing point, as the enthalpy of fusion is directly related to the temperature change during the transition.
Cryoscopy: Unfreezing the Secrets of Solutions
Cryoscopy exploits the phenomenon of freezing point depression to determine the concentration of solutions. When impurities or solutes are dissolved in water, the freezing point is lowered. By accurately measuring the freezing point of a solution, scientists can deduce its concentration. This technique finds applications in analytical chemistry, biochemistry, and even in the food industry for determining the purity and composition of various liquids.
These intricate methods provide scientists with a comprehensive arsenal for measuring the freezing point, a fundamental property that governs countless phenomena in nature and industry. Unraveling the mysteries of freezing point has profound implications for our understanding of thermodynamics, phase changes, and the behavior of matter in diverse environments.