Fermentation: Glycolysis’s Essential Ally For Energy Production In Oxygen-Scarce Environments
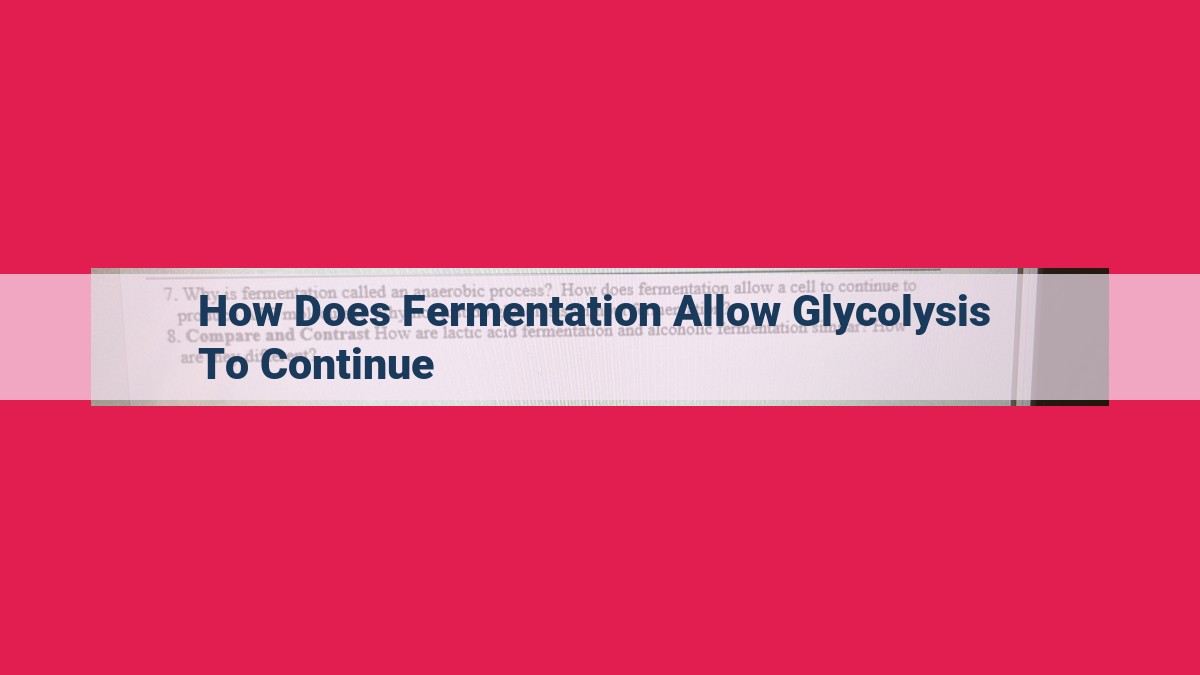
Fermentation allows glycolysis to continue by replenishing NAD+, a cofactor crucial for the process. By reducing pyruvate to lactate (lactic acid fermentation) or ethanol (alcoholic fermentation), fermentation removes pyruvate, preventing its accumulation, which would otherwise inhibit glycolysis. Additionally, fermentation maintains redox balance by producing reducing agents, protecting cells from oxidative damage. This allows glycolysis to continue even in low-oxygen environments, providing energy for cells under anaerobic conditions and ensuring the efficient conversion of glucose into various metabolic products.
Imagine a bustling city, alive with activity. This city represents the glycolysis pathway, a crucial metabolic process that generates energy for our cells. But within this bustling metropolis, a problem lurks: NAD+ depletion.
NAD+ is a vital molecule that acts as an electron carrier in glycolysis. Without enough NAD+, the pathway stalls, halting energy production. Fermentation emerges as the solution, a process that regenerates NAD+ and keeps the energy cycle humming.
Fermentation is a backup plan, employed when oxygen, the preferred electron acceptor for NAD+, is scarce. This anaerobic process allows cells to continue producing energy, ensuring their survival even in challenging environments.
Fermentation: A Dance of Redox and Energy
In the cellular realm, energy production is a constant endeavor. One key player in this dance is glycolysis, a series of reactions that convert glucose into pyruvate, releasing ATP, the energy currency of the cell. However, glycolysis faces a crucial challenge: the depletion of NAD+.
NAD+ (nicotinamide adenine dinucleotide) is an essential electron carrier, acting as a go-between in redox reactions. During glycolysis, NAD+ captures electrons from glucose, becoming reduced to NADH. This depletion of NAD+ would halt glycolysis without timely regeneration.
Enter fermentation, a metabolic tango that replenishes NAD+ to keep the energy party going. Fermentation comes into play when oxygen, the usual electron acceptor in oxidative phosphorylation, is scarce or unavailable.
Two main fermentation pathways exist:
-
Lactate Fermentation: In animals, pyruvate is converted to lactate, a less energetic molecule. This process releases NAD+, allowing glycolysis to continue.
-
Ethanol Fermentation: Yeast and bacteria employ this pathway, converting pyruvate to ethanol and carbon dioxide. This reaction also yields NAD+ and ATP, providing organisms with energy in the absence of oxygen.
Fermentation plays a pivotal role in maintaining the cell’s redox balance. By producing reducing agents such as NADH and ethanol, fermentation protects cells from the damaging effects of reactive oxygen species (ROS). Antioxidants work in tandem with fermentation to neutralize ROS, ensuring a harmonious cellular environment.
In conclusion, fermentation is an ingenious metabolic adaptation that ensures the continuous production of ATP, even in oxygen-limited conditions. By regenerating NAD+ and maintaining redox homeostasis, fermentation empowers cells to thrive in a diverse range of environments. Its significance extends beyond basic cellular functions, finding applications in biotechnology, food production, and the understanding of microbial ecosystems.
Pyruvate: The Roadblock to Glycolysis
Glycolysis, the initial stage of cellular respiration, fuels our cells with energy. But as this process rages on, a byproduct called pyruvate starts accumulating, threatening to halt glycolysis in its tracks.
Pyruvate’s Inhibitory Grip
Imagine pyruvate as a traffic jam on glycolysis’s highway. As it piles up, it slows down the rate of glycolysis, ultimately grinding it to a halt. This would be disastrous for cells, as they rely on this vital pathway for energy production.
Fermentation: The Pyruvate Relief Act
But nature has a clever solution: fermentation. Fermentation is an anaerobic (meaning without oxygen) process that regenerates NAD+ (a crucial electron carrier in glycolysis) and removes pyruvate, allowing glycolysis to continue unchecked.
Lactate Fermentation: The Animal’s Response
In animals, fermentation takes the form of lactate fermentation. Pyruvate is converted into lactate, which accumulates in muscles during intense exercise, causing the familiar burn. Once the exercise is over, the body can reconvert lactate back to pyruvate for further energy production.
Ethanol Fermentation: The Yeast’s and Bacteria’s Choice
Yeast and bacteria employ a different fermentation strategy called ethanol fermentation. Pyruvate is broken down into ethanol (alcohol) and carbon dioxide, releasing energy in the process. This fermentation process is responsible for the production of alcoholic beverages and bread.
Maintaining Redox Balance
Fermentation not only prevents pyruvate accumulation but also contributes to redox balance in cells. By removing pyruvate, fermentation reduces the production of reactive oxygen species (ROS), which can damage cellular components. Fermentation also produces reducing agents that neutralize ROS and protect the cell from oxidative stress.
Fermentation’s Diverse Applications
The applications of fermentation extend beyond energy production and redox balance. It is essential in biotechnology, where microorganisms are used to produce valuable compounds like antibiotics and enzymes. In food production, fermentation is used to create fermented foods like yogurt, cheese, and sauerkraut, which are rich in beneficial bacteria and nutrients.
Fermentation is a crucial metabolic process that plays a pivotal role in glycolysis, pyruvate removal, and redox balance. The two main fermentation pathways, lactate fermentation and ethanol fermentation, are employed by animals, yeast, and bacteria to keep glycolysis running smoothly and protect cells from damage. The diverse applications of fermentation in biotechnology and food production further highlight its importance in our lives.
Fermentation: Maintaining Redox Balance in the Face of Oxidative Stress
Fermentation is a crucial metabolic pathway that allows cells to function without oxygen. One of its important roles is maintaining redox balance, the delicate equilibrium between reducing agents and reactive oxygen species (ROS).
Production of Reducing Agents
During fermentation, reducing agents, such as NADH and FADH2, are produced. These molecules can donate electrons, counteracting the oxidative effects of ROS. By providing a steady supply of reducing power, fermentation helps prevent cellular damage caused by oxidative stress.
Reactive Oxygen Species (ROS) and Redox Balance
ROS, including superoxide and hydrogen peroxide, are natural byproducts of cellular metabolism. In low concentrations, they play a role in signaling and defense. However, excessive ROS can cause oxidative damage to proteins, lipids, and DNA, leading to cell death.
Fermentation as a Shield Against Oxidative Damage
Fermentation protects cells from oxidative damage by:
- Reducing ROS production: By channeling reducing equivalents into fermentation pathways, cells can reduce the production of ROS during metabolism.
- Providing antioxidant defenses: The reducing agents generated during fermentation can directly scavenge ROS, neutralizing their harmful effects.
- Promoting antioxidant enzyme activity: Fermentation also supports the activity of antioxidant enzymes, such as glutathione reductase and superoxide dismutase, which further protect against oxidative stress.
By maintaining redox balance, fermentation allows cells to withstand conditions where oxygen levels are limited or oxidative stress is high. This protective mechanism is crucial for the survival and function of many organisms, including humans.