Unveiling The Power Of False Coloring In Microscopy: Enhancing Contrast And Visual Insights
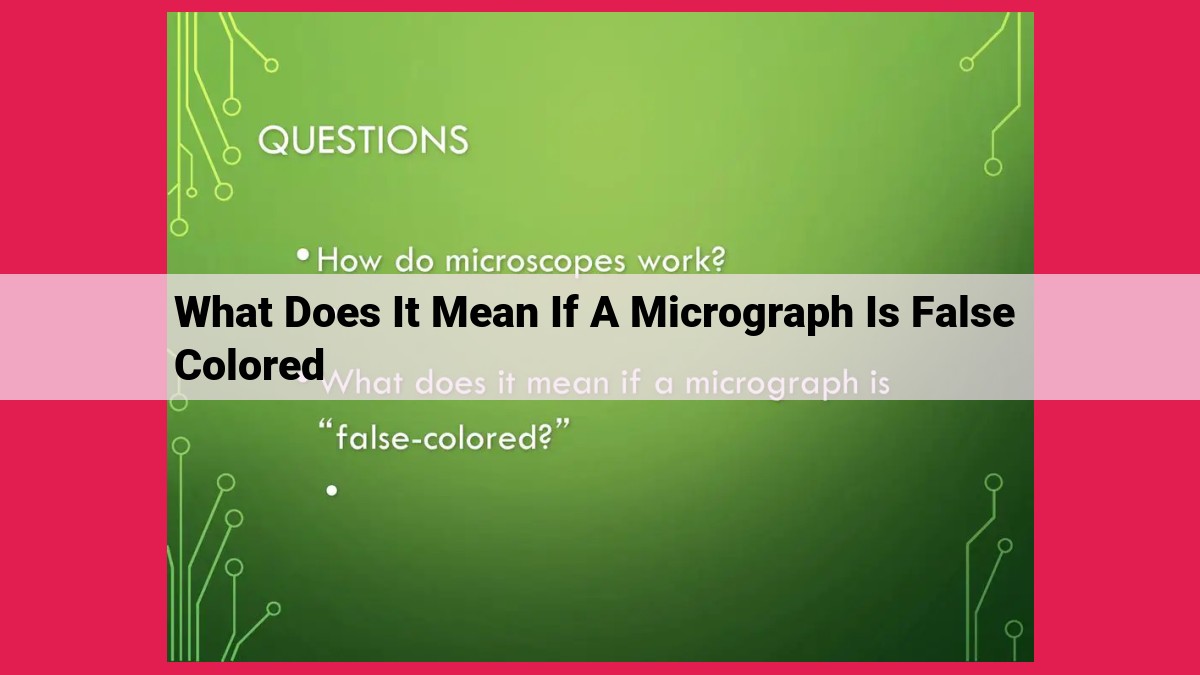
When a micrograph is false colored, its pixels are assigned colors that do not correspond to the actual colors of the sample. This technique is used to enhance contrast, improve resolution, or visualize multiple targets simultaneously in microscopy. False coloring can be achieved through image processing, where color adjustments and enhancements are applied to the micrograph. It is commonly employed in fluorescence microscopy to distinguish between different fluorescent labels, and in other microscopy techniques such as bright-field and electron microscopy. While false coloring can provide valuable insights, it is essential to be aware of potential artifacts and the impact on data quality to avoid misinterpretations.
- Definition of false coloring and its purpose in microscopy.
Unlocking the Secrets of False Coloring in Microscopy: A Journey into Enhanced Visualization
In the realm of microscopy, the intricate world of cells and tissues can be brought into vibrant focus through the use of false coloring. This revolutionary technique goes beyond mere aesthetics to reveal hidden depths of information, enriching our understanding of the microscopic world.
Defining False Coloring: A Colorful Transform
False coloring, also known as pseudocoloring, is the process of assigning colors to microscopic images based on non-color data. This transformation allows us to visualize features and structures that may not be readily apparent in grayscale images. For instance, by assigning green to low-intensity areas and red to high-intensity areas, we can quickly identify regions of high and low activity within a cell.
Purpose of False Coloring: Enhancing the Invisible
The primary objective of false coloring in microscopy is to improve visualization and contrast. By assigning colors that contrast sharply with each other, we can make subtle differences in the image more noticeable. This is particularly useful in distinguishing between closely spaced structures or identifying specific molecules within a sample.
Impact on Microscopy: A Deeper Dive
False coloring has a profound impact on various microscopy techniques. In bright-field microscopy, it enhances the contrast of specimens by assigning colors to different intensities of transmitted light. In dark-field microscopy, it highlights scattered light, making it easier to visualize small particles or fine structures. In electron microscopy, false coloring helps differentiate between different elements or chemical components within a sample.
Beyond Microscopy: Expanding Our Horizons
The power of false coloring extends beyond microscopy. In other scientific disciplines, such as medical imaging and remote sensing, it is used to enhance the visualization of data and identify patterns that may not be obvious in raw images. By unlocking hidden information, false coloring empowers researchers, clinicians, and scientists to make more informed decisions and gain a deeper understanding of the world around them.
Image Processing for False Coloring in Microscopy: Unveiling Hidden Details
False coloring is a powerful technique used in microscopy to enhance the visual representation of specimens. It involves assigning artificial colors to different features in a micrograph, revealing details that may be otherwise difficult to perceive. Image processing plays a crucial role in optimizing micrographs for effective false coloring.
Enhancing Contrast for Improved Visualization
Image processing techniques can be employed to enhance the contrast between different structures in a micrograph. This is particularly useful when the intrinsic contrast is low, making it challenging to distinguish between features. By adjusting the brightness and contrast levels, image processing can manipulate the pixel values to create a more pronounced difference between areas of interest.
Sharpening Images for Resolution Enhancement
Image processing also offers sharpening algorithms that can improve the perceived resolution of micrographs. These algorithms work by accentuating the edges of structures, making them appear more distinct. This enhanced sharpness allows for finer details to be observed, providing a more comprehensive understanding of the specimen.
Background Noise Reduction for Clarity
Background noise can obscure important features in micrographs, making false coloring less effective. Image processing techniques such as noise reduction filters can suppress unwanted noise while preserving the integrity of the original image. By removing background distractions, false coloring can be applied more effectively, resulting in a clearer and more informative representation of the specimen.
Contrast Enhancement with False Coloring: Unlocking Visual Clar _ity_
In microscopy, false coloring is a powerful tool that transforms grayscale images into vibrant visual landscapes, unlocking hidden details and enhancing contrast for exceptional clarity. This technique plays a transformative role in the field of microscopy, allowing scientists and researchers to delve deeper into the microscopic world with unprecedented precision.
False coloring assigns different colors to distinct grayscale values, thereby accentuating subtle variations and highlighting specific features that may otherwise remain unnoticed. With its color-coding capabilities, false coloring emphasizes contrast, creating a more intuitive and visually appealing representation of the microscopic data.
Consider a micrograph of a tissue sample. The grayscale image may reveal only faint outlines and subtle variations in texture. However, by applying false coloring, different cell types, organelles, or molecular structures can be assigned specific hues, creating a vibrant tapestry that illuminates even the most intricate details.
Through its ability to enhance contrast, false coloring empowers researchers to distinguish between objects, identify patterns, and uncover relationships that would be difficult to discern in a grayscale image. It facilitates visualization, enabling scientists to gain deeper insights and draw more accurate conclusions from their microscopic observations.
Resolution Enhancement Through False Coloring
Drawing the line between fact and fiction can be tricky when it comes to microphotography. But there are times when resolution matters, and the ability to visualize the minute details can make all the difference. False coloring comes to the rescue, acting like a superhero, enhancing the perceived resolution of microscopic images.
Imagine your microscope as a window to a miniature world. False coloring is like a special lens that allows you to see more clearly. By assigning different colors to different wavelengths, false coloring highlights specific features, making them stand out from the background noise.
Think of it as painting over a grayscale image. False coloring adds a layer of color and contrast, making it easier to distinguish between subtle variations, precisely locating specific structures. This improved resolution allows scientists to scrutinize and analyze microscopic details with greater accuracy and precision.
So, while false coloring may not alter the physical resolution of an image, it enhances our perception, allowing us to see more, understand more, and unravel the mysteries of the microscopic world.
False Coloring: Enhancing Fluorescence Microscopy for Multi-Target Visualization
In the realm of microscopy, false coloring plays a transformative role, adding a splash of color to micrographs, revealing hidden details and enhancing our understanding of intricate biological structures. This technique is particularly potent when it comes to fluorescence microscopy, allowing researchers to visualize multiple targets simultaneously, expanding the horizons of scientific exploration.
Fluorescence microscopy relies on the emission of fluorescent light by specific molecules labeled with fluorophores. However, the challenge lies in distinguishing between different fluorophores, especially when they emit light in similar wavelengths. False coloring comes to the rescue by assigning different colors to each fluorophore, creating a vibrant visual representation that allows scientists to effortlessly differentiate between distinct targets.
For instance, in studying neuronal circuits, researchers often use fluorescence microscopy to visualize different neuron populations. By employing false coloring, they can assign a blue hue to one neuron population and a green hue to another, making it easy to trace the connections between these neurons and unravel the complexities of the brain’s intricate communication network.
Moreover, false coloring enhances the ability to detect and quantify specific cellular structures. By assigning a distinct color to a particular protein or organelle, researchers can rapidly identify its presence and distribution within cells. This enables them to analyze protein localization patterns, study cellular dynamics, and gain insights into cellular function at a subcellular level.
The power of false coloring in fluorescence microscopy extends beyond simple visualization. By manipulating the assignment of colors, researchers can even enhance the contrast between targets, making it easier to distinguish between closely spaced or overlapping structures. Additionally, false coloring can help to correct for autofluorescence, a background noise that can interfere with the detection of specific targets.
In conclusion, false coloring is an invaluable tool in fluorescence microscopy, providing researchers with the ability to visualize multiple targets simultaneously, enhance contrast, and gain a deeper understanding of cellular structures and processes. By embracing the power of color, scientists can illuminate the hidden complexities of the microscopic world and push the boundaries of scientific discovery.
False Coloring in Microscopy Techniques
Bright-Field Microscopy:
In bright-field microscopy, light illuminates the sample from above and passes through it. False coloring can enhance the contrast between different structures by assigning colors to various shades of gray. For instance, nuclei may be highlighted in blue to distinguish them from the light-colored background.
Dark-Field Microscopy:
In dark-field microscopy, light is scattered by the sample, and only the scattered light is detected. False coloring can be used to visualize small particles or fine structures that may be difficult to see under normal illumination. By assigning vibrant colors to the scattered light, the details can be made more apparent.
Electron Microscopy:
Electron microscopy uses a beam of electrons instead of light to create images. False coloring is often used in electron microscopy to enhance the visibility of different cell components. For example, in transmission electron microscopy (TEM), colors can be assigned to distinguish between organelles, such as mitochondria (purple) and ribosomes (green).
False coloring is a valuable tool in microscopy, allowing researchers to enhance contrast, improve resolution, and visualize multiple targets simultaneously. It plays a significant role in various microscopy techniques, from bright-field to dark-field and electron microscopy, providing deeper insights into cellular structures and functions. However, it’s essential to be aware of the potential artifacts and data quality implications associated with false coloring to use it responsibly and avoid misinterpretations.
Artifacts and False Coloring in Microscopy
False coloring, a powerful tool in microscopy, can also introduce potential artifacts. These artifacts may stem from various factors, including image acquisition, processing, or the interpretation of the resulting images.
One common artifact is color bleed. This occurs when one color channel spills over into another, creating inaccurate representations. To minimize this, advanced image processing algorithms or specific filters can be employed.
Saturation halos can also arise, where bright objects appear surrounded by a halo of saturated color. This artifact can be mitigated by using appropriate color blending techniques or by adjusting the saturation levels.
Another concern is false localization. False coloring can sometimes shift the perceived location of an object, making it appear closer to or farther from its actual position. Careful consideration and validation of the coloring algorithm are crucial to avoid this.
Over-interpretation is a potential pitfall when dealing with false-colored images. It’s essential to remember that these images represent processed data and not absolute truth. Researchers should interpret the findings cautiously, considering the underlying data and any potential artifacts.
To mitigate these artifacts, meticulous image processing techniques, thorough validation, and critical interpretation are paramount. By following best practices and acknowledging the limitations, researchers can harness the benefits of false coloring while minimizing the potential for artifacts and ensuring the accuracy of their observations.
Data Quality and False Coloring: A Cautionary Tale
In the realm of microscopy, false coloring can be a powerful tool to enhance the visualization of microscopic structures. However, it’s crucial to proceed with caution, as false coloring can also introduce artifacts and potentially lead to misinterpretations.
One key consideration is the impact on data quality. False coloring alters the inherent colors of the specimen, which can obscure subtle changes or details that may be important for analysis. It’s essential to carefully evaluate the implications of false coloring on the accuracy and reliability of your data.
Furthermore, responsible use of false coloring is paramount to avoid misinterpretations. By understanding the limitations and potential pitfalls of false coloring, researchers can employ it appropriately and effectively. This includes considering the intended audience and the context in which the images will be presented.
Ultimately, the goal is to communicate scientific findings clearly and accurately. False coloring should be used as a complementary tool to enhance visualization, not as a substitute for careful observation and interpretation of the original micrographs. By adhering to these principles, we can ensure that false coloring serves as a valuable asset in the exploration of the microscopic world while preserving the integrity and reliability of our data.