Factors Influencing Chemical Reaction Rates: A Comprehensive Guide To Enhance Reaction Kinetics
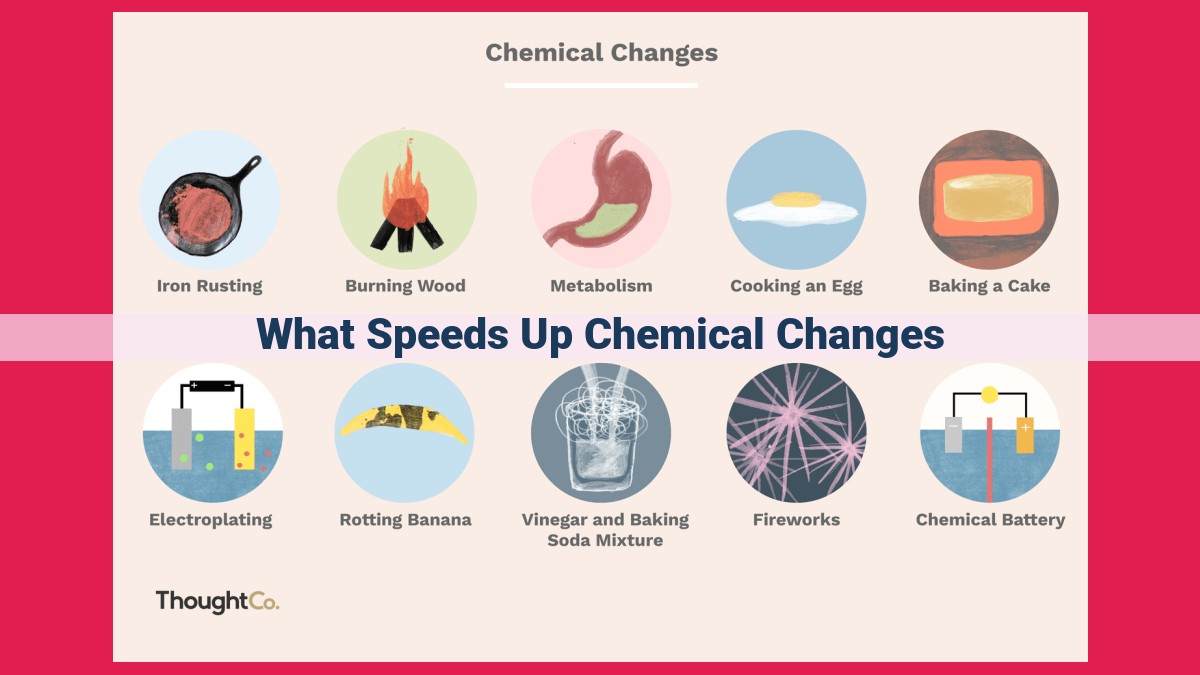
Chemical changes occur faster with increased
- temperature (acceleration of kinetic energy),
- concentration (higher collision probability),
- surface area (enhanced reactant contact),
- catalyst presence (reduced activation energy),
- light energy (excitation of molecules),
- pressure (gas volume, concentration, and temperature changes), and
- solvent polarity (solubility and reaction rate effects).
Temperature: The Heat Catalyst
In the realm of chemistry, reactions occur when molecules collide with enough energy to surmount a barrier called activation energy. It’s like trying to start a bonfire – you need sufficient heat to ignite the kindling.
Temperature plays a crucial role in this process. It’s the measure of kinetic energy – the energy of motion – within molecules. As temperature rises, molecules move faster, increasing the likelihood of effective collisions.
Imagine a room filled with people at a party. The temperature represents the overall excitement level. When the temperature is low, people are more likely to stand around in small groups, making it harder for new connections to form.
But when the temperature rises – say, with some lively music or stimulating conversation – people become more energetic and start moving around more. This increased kinetic energy means they’re more likely to bump into new people, leading to more interactions and, potentially, more chemistry!
So, in chemical reactions, higher temperatures promote faster molecular movement, resulting in more collisions and, ultimately, a quicker reaction rate.
Concentration: The Crowded Dance Floor of Chemical Reactions
Introduction:
In the fast-paced world of chemical reactions, concentration plays a crucial role, acting as a choreographer that orchestrates the dance of molecules and determines the speed at which reactions unfold. Just like a crowded dance floor teems with bodies, a higher concentration of reactants means more molecules jostling and colliding, leading to a flurry of interactions.
Moles and Molarity: Counting the Molecules
The number of molecules in a solution is expressed in units of moles, which is like counting the number of dancers on the dance floor. Molarity, on the other hand, measures the concentration of reactants, which is analogous to the number of dancers per unit area. A higher molarity means more molecules packed into a given space, resulting in a more crowded dance floor and increased chances of collisions.
Volume: Expanding the Dance Floor
The volume of a solution serves as the venue for the dance, influencing the number of molecules present. Think of it as expanding or shrinking the dance floor, directly affecting the concentration. A larger volume dilutes the reactants, spreading them out like dancers on a vast dance floor, while a smaller volume concentrates them, bringing them closer together and amplifying the collision frequency.
Real-World Examples
In daily life, we encounter numerous examples of the impact of concentration. The faster a piece of meat cooks in a high-concentration marinade compared to a low-concentration one is a testament to the role of concentration. Likewise, the efficacy of cleaning agents depends on the concentration of active ingredients, with higher concentrations ensuring more effective removal of dirt and grime.
Conclusion:
Concentration, the choreographer of chemical reactions, orchestrates the dance of molecules and governs the speed of reactions. By understanding the interplay between moles, molarity, and volume, we gain insights into the dynamics of chemical interactions, empowering us to control and optimize these reactions for various applications in science, industry, and everyday life.
Surface Area: More Surface, More Reactions
Imagine a crowded dance floor. The more people there are, the more likely they are to bump into each other. The same principle applies to chemical reactions: the more reactant molecules there are in contact, the more likely they are to react.
Surface area is a measure of how much of a substance is exposed to its surroundings. It’s like the skin of a ball: the larger the ball, the more skin it has. In a chemical reaction, the surface area of the reactants is crucial.
The smaller the particle size, the larger the surface area. Think of it this way: if you cut a ball into smaller and smaller pieces, each piece will have more skin than the original ball. The same is true for reactants: smaller particles have more surface area, meaning more reactant molecules can come into contact and react.
The shape of the particles also affects the surface area. A cube has six faces, so it has less surface area than a sphere with the same volume. This is because the sphere’s curved surface exposes more of the reactant molecules.
Porosity is another factor that can increase surface area. Porous materials have tiny holes or pores, which increase the surface area available for reactions to take place. Activated carbon, for example, is a highly porous material used in water filters and gas masks to increase the surface area for adsorption and chemical reactions.
By increasing the surface area of reactants, you can increase the reaction rate. This is important in a variety of applications, such as catalysis, drug delivery, and food processing. By understanding the role of surface area in chemical reactions, scientists and engineers can design and optimize reactions for a wide range of purposes.
Catalysts: The Helping Hand
- Introduce enzymes and their role in providing alternative reaction pathways, lowering activation energy, and speeding up reactions.
Catalysts: The Orchestrators of Chemical Reactions
In the realm of chemistry, reactions often rely on a helping hand to ignite their transformative power. Catalysts, like skilled conductors, orchestrate these reactions, enabling them to proceed at an accelerated pace.
Enzymes: Nature’s Precision Tools
Enzymes, the maestros of the biological world, are intricate protein molecules. They possess an uncanny ability to facilitate specific chemical reactions while remaining unchanged themselves. Enzymes work by providing alternative pathways for reactions to occur, lowering the activation energy required to initiate the process.
Lowering Activation Energy: A Path of Least Resistance
Activation energy is the initial energy barrier that must be overcome for a reaction to proceed. Enzymes, with their molecular ingenuity, offer a path of least resistance, reducing this energy barrier and allowing reactions to transpire with greater ease.
Speeding Up Reactions: A Symphony of Efficiency
As the activation energy is lowered, the frequency of effective collisions between reactants increases, leading to a dramatic acceleration of the reaction rate. Enzymes act as catalysts in this symphony of efficiency, orchestrating reactions with exceptional speed and precision.
Light Energy: The Illuminating Force in Chemical Reactions
In the magical realm of chemistry, certain reactions require a spark, a touch of illumination, to ignite their dance. Enter light energy, a powerful force that can alter the fate of molecules, orchestrating extraordinary transformations.
When light strikes a molecule, its energy can resonate with specific wavelengths, creating a state of excitement. This excitation disrupts the molecule’s equilibrium, propelling its electrons to higher energy levels. It’s like a cosmic ballet, with electrons twirling and swirling, energized by the light’s embrace.
This newfound energy grants the molecule a burst of reactivity. It becomes more prone to react with other molecules, as if the light has illuminated a hidden pathway to chemical interaction. Imagine a shy dancer suddenly finding the courage to step into the spotlight, ready to seize the moment.
For some reactions, light energy acts as a catalyst, lowering the activation energy required for the transformation to occur. It’s like a gentle hand guiding the reactants along a smoother path, making their encounter more likely. In other cases, light energy can even initiate reactions that would otherwise be impossible in the dark.
The wavelength of the light plays a crucial role in this molecular dance. Different wavelengths correspond to specific energies, which can selectively excite different types of molecules. It’s like having a symphony of light, each note resonating with a different chemical partner.
So next time you’re marveling at a blooming flower or the vibrant colors of a sunset, remember that light is not merely an aesthetic phenomenon. It’s a powerful force that shapes the world around us, illuminating the path of chemical reactions and igniting the spark of life itself.
Pressure: Squeezing for Speed
Picture a crowded dance floor, teeming with people. As the night wears on, the room fills to capacity, creating a sense of claustrophobia. Just as the crowd’s proximity affects their movements, so too does pressure influence the speed of chemical reactions.
In a gas-phase reaction, pressure exerts a significant effect on the volume, concentration, and temperature of the reactants. Let’s delve into each of these factors:
-
Volume: As pressure increases, the volume of the gas decreases. This compresses the reactants, increasing their proximity. With more molecules crammed into a smaller space, they have a greater chance of colliding and reacting.
-
Concentration: As the volume decreases, the concentration of the reactants surges. This increase in concentration means there are more reactants packed into the same space, heightening the probability of successful collisions.
-
Temperature: Pressure changes can also affect temperature. When a gas is compressed, its molecules move more rapidly due to the increased collision frequency. This increase in kinetic energy enhances the molecules’ reactivity, speeding up the reaction rate.
So, by squeezing the reactants closer together and boosting their energy, pressure acts as a potent catalyst. It accelerates the dance of chemical reactions, leading to faster outcomes.
Remember, however, that pressure’s influence is context-specific. For some reactions, increased pressure may have little or no effect. Nonetheless, for gas-phase reactions, pressure remains a crucial factor in dictating the pace of chemical transformations.
Solvent Effects: The Chemical Bath
The Role of Solvents
In chemical reactions, solvents play a pivotal role as the medium in which the reactants dissolve and interact. The nature of the solvent can significantly influence the solubility, reactivity, and overall reaction rates.
Solvent Polarity
The polarity of a solvent, measured by its dipole moment, refers to the uneven distribution of electrons within its molecules. Polar solvents, such as water, have a significant separation of positive and negative charges, while nonpolar solvents, such as hexane, have a more uniform distribution.
Solubility of Solutes
The polarity of the solvent directly affects the solubility of solutes. Like dissolves like, meaning polar solvents dissolve polar solutes well, while nonpolar solvents dissolve nonpolar solutes well. This principle is crucial for choosing the appropriate solvent for a particular reaction or extraction process.
Hydrogen Bonding
Hydrogen bonding is a type of strong intermolecular attraction that occurs when a hydrogen atom is covalently bonded to a highly electronegative atom, such as oxygen, nitrogen, or fluorine. Solvents that can form hydrogen bonds, such as water and alcohols, have a significant impact on reaction rates.
Influence on Reaction Rates
Solvent effects on reaction rates are multifaceted. Polar solvents can solvate ions and polar molecules more effectively, leading to increased electrostatic interactions and faster reactions. Conversely, nonpolar solvents can promote reactions between nonpolar molecules by reducing the solvation effect.
Examples
The influence of solvent effects can be observed in various reactions. For example, in the SN2 nucleophilic substitution reaction, polar solvents like water promote the reaction by stabilizing the transition state, while nonpolar solvents like dimethylformamide (DMF) slow down the reaction. In the Diels-Alder cycloaddition reaction, polar solvents enhance the reactivity of electron-withdrawing dienophiles, while nonpolar solvents favor electron-donating dienophiles.
Understanding the effects of solvents on chemical reactions is essential for optimizing reaction conditions, predicting outcomes, and designing efficient synthetic strategies. By carefully selecting solvents based on their polarity and hydrogen-bonding abilities, chemists can harness their influence to control reaction rates and achieve desired outcomes.