Determine Extinction Coefficient: Beer’s Law, Molar Absorptivity For Compound Quantification
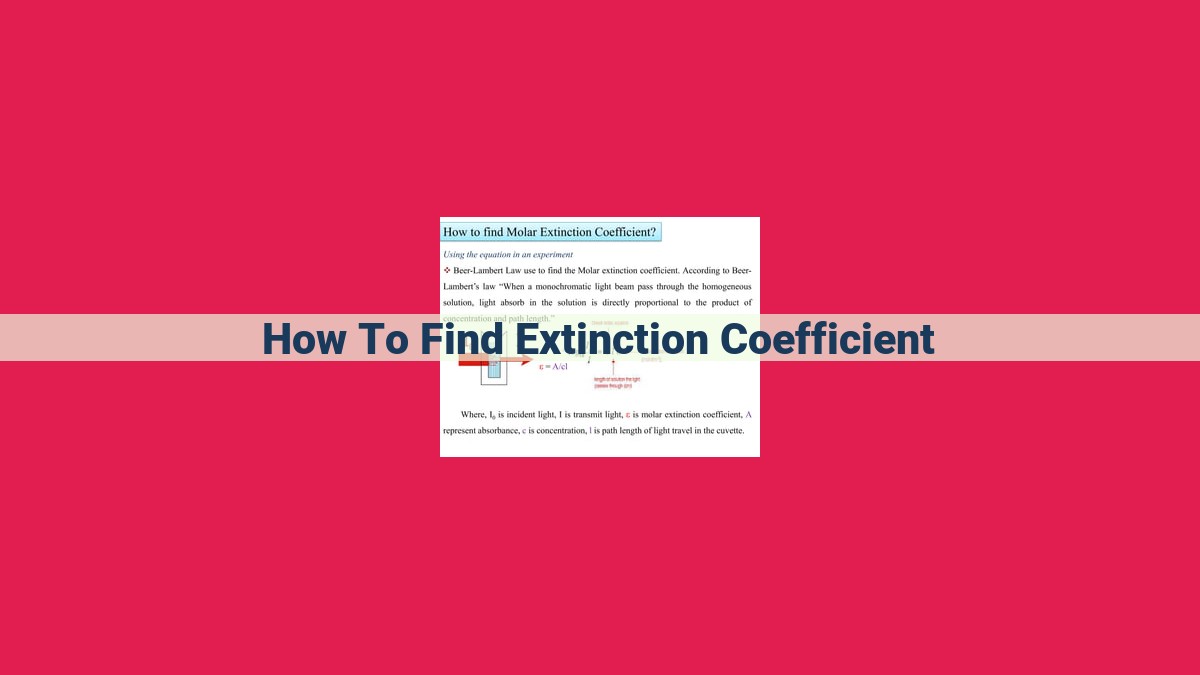
To determine the extinction coefficient, Beer’s law forms the foundation, linking transmittance and absorbance. Absorbance, a measure of light absorption, is proportional to concentration and the molar absorptivity, a compound-specific constant. Using standard curves involving regression analysis, the molar absorptivity is calculated. This coefficient enables scientists to characterize and quantify compounds in a wide array of applications, including biochemistry, environmental monitoring, and analytical chemistry.
Extinction Coefficient: Unraveling the Secrets of Light Absorption and Transmission
In the realm of light’s interaction with matter, extinction coefficient holds a pivotal position, shedding light on the intricate dance between light absorption and transmission. This enigmatic coefficient, often represented by the Greek letter ε, quantifies the ability of a substance to absorb or scatter light. It serves as a crucial tool for scientists and researchers seeking to understand the optical properties of materials and delve into the mysteries of light’s journey through various mediums.
The significance of extinction coefficient lies in its ability to reveal the intricate interplay between light and matter. It unveils the degree to which a substance intercepts light’s path, providing insights into the molecular structure and composition of the material. This knowledge is invaluable in diverse fields, from biochemistry to environmental monitoring and analytical chemistry.
Imagine a beam of light embarking on a journey through a substance. As it traverses this medium, some of its photons encounter molecules and atoms, resulting in absorption or scattering. The extinction coefficient quantifies the extent of this interaction, measuring the fraction of light that is either absorbed or scattered per unit pathlength through the substance. This information unveils the absorptivity and scattering properties of the material, painting a detailed picture of its optical characteristics.
Beer’s Law: The Fundamental Equation
In the realm of light and its interactions with matter, Beer’s Law stands as a cornerstone principle. It’s an equation that unveils the intricacies of how light absorption and transmission unfold. As a scientist or researcher, understanding Beer’s Law empowers you to unravel the mysteries of light’s behavior in various substances.
Transmittance: Unveiling Light’s Penetration
Imagine a beam of light, its photons eagerly marching through a sample. Transmittance measures how much of this light emerges victoriously on the other side. It quantifies the fraction of light that successfully navigates the substance, providing insights into the material’s transparency. A high transmittance indicates a material that allows light to pass through effortlessly, while a low transmittance reveals a more opaque nature.
Absorbance: Quantifying Light’s Capture
While transmittance reveals how much light slips through, absorbance unveils the amount of light that succumbs to the substance’s embrace. Visualize photons, like tiny dancers, gracefully swaying and then suddenly being absorbed by the atoms and molecules within the sample. Absorbance measures the extent to which a substance intercepts and traps light, giving scientists a glimpse into the material’s ability to absorb特定 wavele ngths.
Molar Absorptivity: A Constant of Proportionality
Molar absorptivity, a material-specific constant, enters the scene as the proportionality factor. It establishes the unique relationship between a substance’s concentration and its absorbance. Amazingly, this constant holds valuable information about the compound, allowing scientists to identify and quantify specific substances within a sample.
The Intimate Relationship Between Transmittance and Absorbance: Unraveling the Secrets of Light Interaction
In our exploration of extinction coefficient, we delve into the fascinating relationship between transmittance and absorbance, two crucial measures that illuminate the behavior of light as it interacts with matter. This relationship is elegantly captured in the renowned Beer’s Law equation, a cornerstone of analytical chemistry.
Transmittance quantifies the amount of light that successfully navigates through a sample, offering insights into its penetrability. Its counterpart, absorbance, measures the fraction of light absorbed by the sample, providing clues about the extent of its interaction.
The essence of Beer’s Law lies in establishing a direct connection between these two parameters. The equation succinctly states that absorbance (A) is directly proportional to the concentration (c) of the absorbing substance in the sample, the path length (l) through which the light travels, and a constant value known as molar absorptivity (ε).
Mathematically, this relationship is expressed as:
A = ε * c * l
This equation forms the foundation for quantitative analysis. By precisely measuring absorbance, scientists can determine the concentration of an unknown substance, provided they know the values of molar absorptivity and path length, which are typically determined experimentally.
The molar absorptivity is a unique property of each compound, representing the amount of light absorbed per mole of substance per unit of path length. Armed with this knowledge, scientists can identify compounds by matching their molar absorptivity against reference values.
Molar Absorptivity: The Fingerprint of Compounds
In the realm of light absorption and transmission, extinction coefficient plays a pivotal role in understanding the behavior of light as it interacts with matter. Among the different components of extinction coefficient, molar absorptivity stands out as a unique property that offers invaluable insights into the identity and concentration of compounds.
Defined as the proportionality constant in Beer’s Law equation, molar absorptivity represents the amount of light absorbed by a substance per unit path length and concentration. Measured in units of (M^{-1}cm^{-1}), it is a characteristic property that distinguishes one compound from another.
The role of molar absorptivity extends beyond mere identification. It serves as a crucial tool in determining the concentration of a compound in a sample. By measuring the absorbance of light at a specific wavelength and comparing it to a standard curve, scientists can accurately quantify the concentration of the analyte.
The uniqueness of molar absorptivity stems from its specificity for each compound. This property enables scientists to identify and distinguish between different substances, even in complex mixtures. Its predictive nature allows researchers to design experiments and analytical methods tailored to specific compounds.
Molar absorptivity finds widespread applications in various fields, including biochemistry, environmental monitoring, and analytical chemistry. It is a versatile parameter that empowers scientists to explore the molecular makeup of materials, monitor environmental contaminants, and analyze complex chemical systems with precision and accuracy.
Calibration with Standard Curves
Setting the Stage
Precision is paramount in scientific measurements, and when it comes to determining the concentration of a particular substance, calibration with standard curves is an indispensable technique. It’s like having a reliable roadmap that guides you to accurate results.
The Significance of Standard Curves
Standard curves are the unsung heroes of concentration measurements. They provide a crucial reference point that allows you to translate absorbance values into precise concentration values. By plotting known concentrations of a substance against their corresponding absorbance values, you create a graphical representation that serves as a guide for determining the concentration of unknown samples.
The Alchemy of Regression Analysis
To transform the standard curve into a quantitative tool, regression analysis steps into the picture. This mathematical technique identifies the best-fit line that describes the relationship between concentration and absorbance. The equation of this line becomes the heart of your calibration curve, allowing you to calculate unknown concentrations with ease.
Harnessing the Standard Curve
With the calibration curve in hand, you now have a powerful weapon in your analytical arsenal. Simply measure the absorbance of your unknown sample, plug it into the calibration equation, and presto! You have a precise concentration value. It’s like having a secret formula that unlocks the mysteries of unknown substances.
Applications in the Real World
The versatility of calibration curves extends far beyond the walls of the laboratory. They find widespread application in fields as diverse as biochemistry, environmental monitoring, and analytical chemistry. From determining the concentration of proteins in a biological sample to measuring the levels of pollutants in water, calibration curves play a crucial role in ensuring the accuracy of scientific measurements.
Unveiling the Applications of Extinction Coefficient
The extinction coefficient, a fundamental concept in optics and spectroscopy, finds widespread applications in diverse scientific fields. Its significance lies in its ability to quantify the interaction between light and matter, providing valuable insights into molecular structure, concentration, and behavior.
In biochemistry, the extinction coefficient plays a crucial role in spectrophotometry, a technique used to determine the concentration of molecules in a sample. By measuring the absorbance of light at a specific wavelength, scientists can use Beer’s Law to calculate the concentration of the analyte of interest. This technique is commonly employed in DNA quantification, protein assays, and enzyme assays, providing essential information for research and diagnostics.
In environmental monitoring, the extinction coefficient is utilized to assess the turbidity of water bodies, a measure of the amount of suspended particles. This data aids in evaluating water quality and identifying potential contaminants. Moreover, it helps monitor the effectiveness of water treatment processes and protect aquatic ecosystems.
In analytical chemistry, the extinction coefficient serves as a fingerprint for different compounds, enabling their identification and quantification. By measuring the absorbance of a sample at multiple wavelengths, scientists can create a unique spectral fingerprint that can be compared to reference data to determine the identity and concentration of unknown compounds. This technique is widely used in pharmaceutical analysis, forensic science, and food chemistry.
Additionally, the extinction coefficient finds applications in fields such as astronomy, where it helps determine the composition and distance of celestial objects, and in materials science, where it aids in understanding the optical properties of thin films and nanomaterials.
The versatility and significance of the extinction coefficient make it an indispensable tool in scientific research and practical applications. It empowers scientists to unravel the secrets of molecular structure, monitor environmental health, identify unknown compounds, and explore the vast universe.