Enzyme Regulation: Mechanisms For Precise And Timely Control In Metabolism
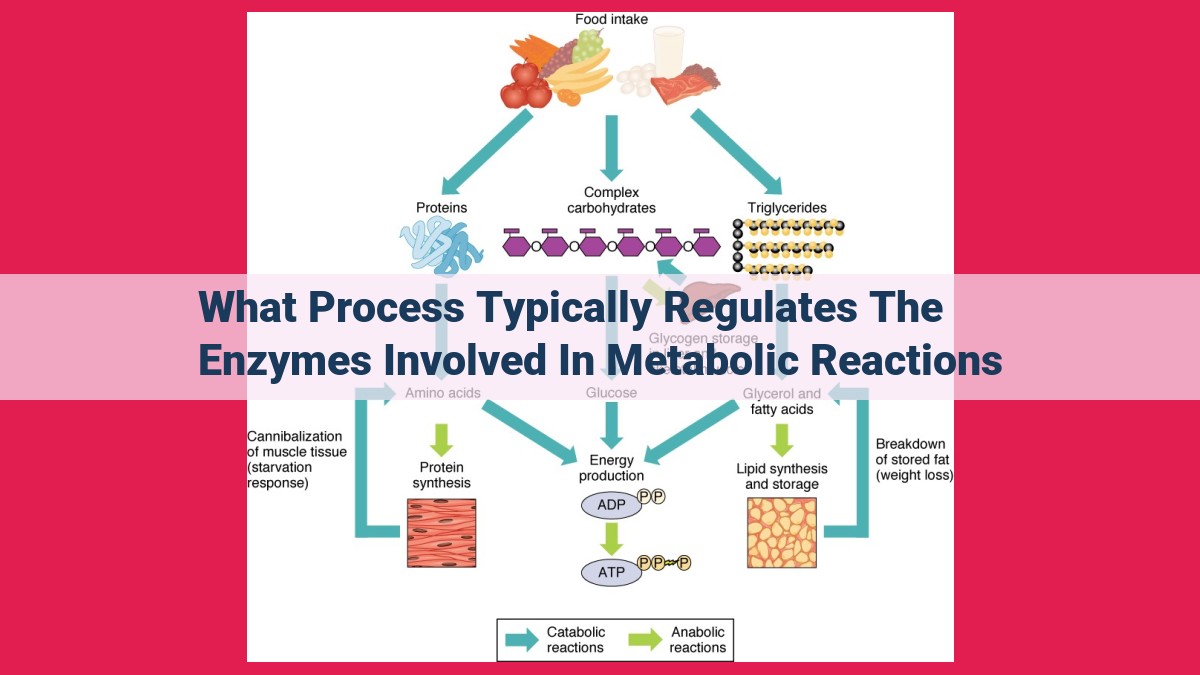
Enzyme activity in metabolic reactions is tightly controlled through various mechanisms, including feedback inhibition, allosteric regulation, covalent modifications, and gene expression. Feedback inhibition prevents overproduction by inhibiting enzymes that catalyze early steps in a pathway when end-products accumulate. Allosteric regulation involves non-substrate molecules that bind to enzymes and influence their activity. Covalent modifications alter enzyme structure and activity, while gene expression regulates enzyme production. These mechanisms work in concert to maintain cellular homeostasis by ensuring precise and timely enzyme function.
Unveiling the Masterful Catalysts: Enzymes and Metabolic Reactions
In the bustling metropolis of our cells, countless chemical reactions occur, each playing a pivotal role in maintaining life. But behind the scenes, there lies a silent army of master catalysts that make these reactions possible: enzymes.
Enzymes: The Tiny Champions of Chemical Transformations
Enzymes, nature’s chemical magicians, are proteins that facilitate chemical transformations without being consumed in the process. They act as the spark plugs for cellular reactions, lowering the activation energy required for them to occur. Without these enzymatic allies, our metabolic machinery would grind to a halt.
How Enzymes Work Their Magic
Each enzyme possesses a unique active site, a specialized region that selectively binds to and orients the reactant molecules to facilitate their interaction. This precise alignment lowers the activation energy, making the reaction more likely to proceed.
The Interplay of Enzymes and Metabolism
The activity of enzymes is tightly regulated to ensure that metabolic reactions occur at the optimal rate. This regulation can take various forms, including:
-
Feedback Inhibition: When the end-product of a metabolic pathway accumulates, it can bind to the enzyme responsible for its production, inhibiting its activity and preventing overproduction.
-
Allosteric Regulation: Non-substrate molecules, known as allosteric effectors, can bind to enzymes and influence their activity. These effectors can either activate or inhibit the enzyme, modulating its function.
-
Covalent Modification: Enzymes can undergo covalent modifications, such as phosphorylation or glycosylation, which can alter their structure, activity, and stability.
Feedback Inhibition: A Tale of Metabolic Harmony
In the bustling city of metabolism, enzymes play the role of tireless workers, catalyzing countless chemical reactions that keep the cellular machinery humming. However, to maintain order amid the chaos, cells have devised a clever regulatory mechanism known as feedback inhibition.
Envision a busy assembly line, where enzymes toil away, churning out end-products like widgets. Feedback inhibition is like a vigilant supervisor, keeping an eagle eye on the end-product levels. When the widgets pile up, this supervisor steps in and whispers to the enzymes, “Enough, my friends. We’ve got all we need.”
How does feedback inhibition work? It’s a tale of molecular messengers and chemical “off switches.” The end-product itself acts as the messenger, binding to a specific site on the enzyme responsible for its production. This binding triggers a conformational change, like a key fitting into a lock. The result? The enzyme’s activity is turned off, preventing the further production of that end-product.
This mechanism ensures that cellular metabolism remains in delicate balance, avoiding both product shortages and surpluses. It’s like a symphony conductor, orchestrating the flow of reactions to meet the cell’s exact needs. By finely tuning enzyme activity, feedback inhibition ensures that metabolic reactions proceed in an orderly and efficient manner.
However, this regulatory mechanism is not merely reactive. Cells can also anticipate end-product accumulation and proactively adjust enzyme activity through feedback inhibition. This ensures a smooth transition between metabolic pathways, preventing abrupt shifts in the production of essential molecules.
In the grand scheme of cellular life, feedback inhibition plays a pivotal role in maintaining metabolic homeostasis. It’s a testament to nature’s ingenuity, showcasing the intricate control mechanisms that govern our bodies’ microscopic machinery.
Allosteric Regulation: The Magic of Non-Substrate Molecules
Enzymes, the workhorses of our cells, meticulously orchestrate chemical reactions that sustain life. But how do cells control these enzymatic maestros to ensure their actions are in harmony with the body’s needs? One key regulatory mechanism is allosteric regulation, where non-substrate molecules act as puppeteers, influencing enzyme activity.
Imagine an enzyme as a finely tuned machine, with a specific substrate binding to a designated site, the “active site.” While this substrate-enzyme interaction is crucial for kicking off the chemical transformation, other molecules can also bind to the enzyme, influencing its behavior. These non-substrate molecules, known as allosteric effectors, act like remote controls, either boosting or dampening enzyme activity.
Positive allosteric effectors are like cheerleaders, giving the enzyme a helping hand. They enhance the enzyme’s affinity for its substrate, increasing reaction rates. On the flip side, negative allosteric effectors act as brakes, weakening the enzyme’s grip on its substrate and slowing down the reaction.
This allosteric regulation plays a vital role in fine-tuning metabolism. Take the example of the enzyme aspartate transcarbamoylase (ATCase), a key player in synthesizing nucleotides. When levels of nucleotides are high, CTP, a nucleotide, acts as a negative allosteric effector for ATCase, putting the brakes on the reaction and preventing overproduction of nucleotides.
Therefore, allosteric regulation is a remarkable mechanism that allows cells to precisely control enzymatic activity, ensuring that metabolic pathways run smoothly, responding to changes in cellular conditions and maintaining a harmonious balance within our bodies.
Covalent Modifications: Shaping Enzyme Function
In the bustling city of metabolism, enzymes play the role of skilled craftsmen, meticulously orchestrating intricate chemical reactions. But how do cells ensure these enzymes work in harmony? One ingenious strategy is covalent modification, a process that involves attaching or removing chemical groups to the enzyme’s amino acid building blocks.
Phosphorylation, a common covalent modification, resembles a molecular switch. When a phosphate group is attached to a specific amino acid, it can activate the enzyme, turning it into a metabolic powerhouse. Conversely, removing the phosphate group inactivates the enzyme, allowing the cell to fine-tune its metabolic output.
Methylation is another chemical dance that can alter enzyme activity. By adding a methyl group (a carbon with three hydrogen atoms) to an amino acid, methylation can either enhance or diminish the enzyme’s power. It’s like a dimmer switch, adjusting the enzyme’s influence on metabolic pathways.
Glycosylation, the attachment of sugar molecules to amino acids, often increases enzyme stability. Sugars act as a protective shield, preventing the enzyme from degradation and extending its lifespan. This modification is particularly crucial for enzymes that reside in harsh cellular environments.
Ubiquitination, a more complex covalent modification, involves attaching a small protein called ubiquitin. It serves as a signal for the cell’s recycling system, marking the enzyme for degradation. This process helps eliminate damaged or unnecessary enzymes, ensuring cellular homeostasis.
Covalent modifications are a testament to the cell’s remarkable ability to fine-tune its metabolic machinery. By dynamically altering enzyme structure, activity, and stability, cells can orchestrate complex metabolic processes with precision and efficiency. These modifications are essential for a healthy and functioning cellular environment. In the ever-changing landscape of cellular metabolism, covalent modifications take center stage as essential regulators, shaping enzyme function and maintaining cellular balance.
Gene Expression: Regulating Enzyme Production
In the intricate dance of cellular metabolism, enzymes play pivotal roles as the catalysts for countless chemical transformations. Their production, however, is not haphazard; it is meticulously regulated by a masterful conductor known as gene expression.
Gene expression controls the synthesis of enzymes by translating genetic information into functional proteins. This intricate process involves several key steps:
-
Transcription: DNA, the blueprint of life, is transcribed into messenger RNA (mRNA), a molecular intermediary.
-
Translation: Ribosomes, the cellular protein factories, decipher the mRNA code and assemble amino acids into enzyme polypeptides.
The rate of gene expression, and hence enzyme production, is influenced by a symphony of factors:
-
Environmental cues: External stimuli, such as nutrient availability or hormones, can trigger signaling pathways that activate or repress gene expression.
-
Transcription factors: Specialized proteins bind to specific DNA sequences and either enhance or suppress transcription.
-
Epigenetic modifications: Chemical tags on DNA or histones, the proteins that package DNA, can alter gene accessibility and expression.
By modulating gene expression, cells can fine-tune enzyme production to meet the ever-changing demands of metabolism. This regulatory prowess is essential for maintaining cellular homeostasis and orchestrating the intricate symphony of life.
Interrelation of Regulatory Mechanisms
The intricate web of enzyme regulation extends beyond individual mechanisms. These mechanisms intertwine, forming a complex network that ensures precise control over cellular metabolism.
For instance, feedback inhibition serves as a watchdog, monitoring the levels of end-products. When levels reach a certain threshold, feedback inhibition kicks in, curbing enzyme activity and preventing overproduction.
Allosteric regulation provides a more flexible means of control. Non-substrate molecules can bind to specific sites on enzymes, modulating their activity. These effectors can either stimulate or inhibit enzymatic reactions, depending on the specific enzyme and effector combination.
Covalent modifications add another layer of complexity. Phosphorylation, for example, can dramatically alter enzyme structure and activity. These modifications can be reversible or irreversible, providing long-term or short-term control, respectively.
Finally, gene expression acts as the master regulator of enzyme abundance. Environmental cues and cellular signals can influence gene expression, dictating the amount and type of enzymes produced. This global regulatory mechanism complements the more localized controls of enzyme activity.
In summary, the interconnection of enzyme regulation mechanisms allows cells to finely tune their metabolism. Feedback inhibition, allosteric regulation, covalent modifications, and gene expression work in concert to ensure that enzymatic reactions proceed at the optimal rate, maintaining cellular homeostasis and proper physiological function.