Enzyme Denaturation: Understanding Protein Structure And Catalytic Activity
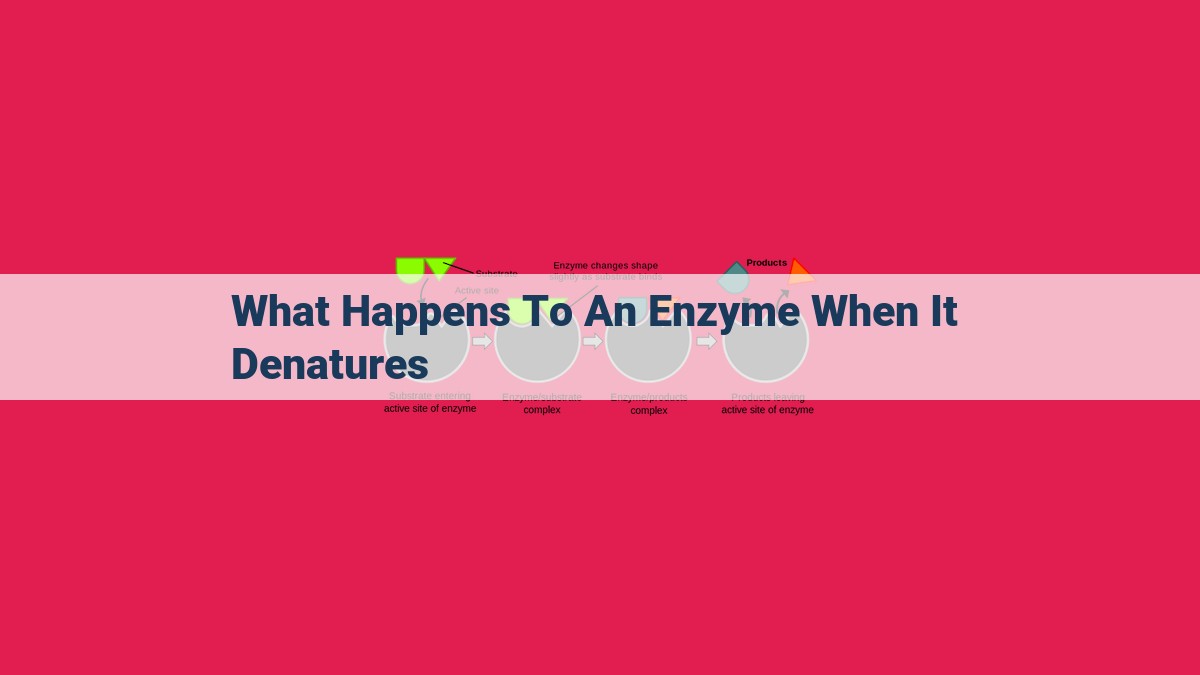
Enzyme denaturation occurs when an enzyme loses its catalytic activity due to changes in its shape and structure. This can happen through various mechanisms, such as unfolding of protein chains, disruption of weak bonds, aggregation, and exposure of hydrophobic groups. Denaturation alters the enzyme’s tertiary and quaternary structure, hindering its ability to interact with substrates and cofactors effectively. The denaturation process can be irreversible, as the enzyme may not be able to regain its native conformation due to the extensive changes in its shape and aggregation.
What is Enzyme Denaturation?
- Definition of denaturation and its impact on enzyme function.
Enzyme Denaturation: Unraveling the Enigma of Enzyme Deactivation
What is Enzyme Denaturation?
In the realm of biochemistry, enzymes reign supreme as the catalysts that orchestrate countless reactions within living organisms. However, under certain conditions, these pivotal biomolecules can undergo a transformative process known as denaturation. This irreversible phenomenon wreaks havoc upon enzymes, annihilating their ability to perform their designated roles within the intricate tapestry of life.
The Mechanisms of Denaturation
Denaturation arises when enzymes are subjected to extreme temperature, pH, or chemical agents. These environmental stressors attack the protein structure of enzymes, causing them to unravel and lose their native conformation. This conformational change is akin to a crumpled origami crane; the once-precise folds and intricate shapes that define an enzyme’s function are now lost, rendering it inactive.
Mechanism of Denaturation
- Loss of catalytic activity due to changes in shape, protein chain unfolding, and aggregation.
Mechanism of Enzyme Denaturation: Unveiling the Destruction of Enzyme Activity
Introduction:
Enzymes, the workhorses of biological processes, orchestrate the seamless flow of chemical reactions that sustain life. However, under certain circumstances, these molecular maestros can encounter a fate known as denaturation, which irreversibly cripples their ability to perform their vital tasks.
Step 1: Loss of Shape
The mechanism of enzyme denaturation begins with changes in the enzyme’s shape. Heat, pH extremes, and certain chemicals can disrupt the delicate scaffold of bonds that hold the enzyme in its precise tertiary and quaternary structure. As a result, the enzyme loses its native conformation, the specific shape that enables it to bind with its target substrate and catalyze its transformation.
Step 2: Unfolding of Protein Chains
The loss of shape triggers the unfolding of protein chains. The enzyme’s polypeptide backbone begins to unravel, causing a loss of the organized tertiary and quaternary structures. This unfolding process exposes previously buried hydrophobic groups to the aqueous environment, leading to further instability and aggregation.
Step 3: Aggregation
As the unfolded protein chains continue to interact with the solvent, they begin to aggregate, forming insoluble clumps. This aggregation further exposes hydrophobic groups, leading to a net charge change on the enzyme’s surface. The reduced solubility of the aggregated enzyme hampers its ability to interact with its substrate, hindering its catalytic activity.
Consequences of Denaturation:
Denaturation marks the irreversible demise of enzyme function. The loss of catalytic activity can have far-reaching consequences for cellular processes and overall organismal health. Even partial denaturation can significantly impair enzyme performance, disrupting metabolic pathways and cellular homeostasis.
Altered Shape of the Enzyme
- Unfolding of protein chains resulting in loss of tertiary and quaternary structure.
- Exposure of hydrophobic groups to the aqueous environment.
Altered Shape of the Enzyme: A Tale of Unfolded Chains
Enzymes are the tireless workers of our cells, facilitating essential chemical reactions. However, under certain conditions, these molecular machines can undergo a dramatic transformation known as denaturation. This process alters the enzyme’s delicate shape, impairing its ability to perform its vital functions.
One key aspect of denaturation involves the unfolding of protein chains. Enzymes are composed of complex chains of amino acids that fold into intricate three-dimensional structures. This intricate origami is essential for maintaining the enzyme’s active site, the molecular pocket where chemical reactions take place. During denaturation, the protein chains lose their carefully orchestrated shape, resulting in a loss of tertiary and quaternary structure.
This structural disruption has far-reaching consequences. With the active site deformed, the enzyme can no longer bind to its substrate molecules and facilitate reactions. Additionally, the unfolding of protein chains exposes hydrophobic groups that were previously shielded within the enzyme’s interior. These hydrophobic groups are repelled by water, the primary component of our bodily fluids. As they are exposed, they seek refuge by clumping together*, forming **aggregates. These aggregates further hinder the enzyme’s ability to function and can ultimately lead to its complete inactivation.
Unfolding of Protein Chains: The Unraveling of Enzyme Structure
In the intricate world of enzymes, denaturation lurks as a formidable foe, disrupting their delicate structure and crippling their catalytic prowess. At the heart of this destructive process lies the unraveling of protein chains, a phenomenon that unfolds like a tragic tale of molecular disarray.
As enzymes perform their vital biochemical tasks, their protein chains are held together by a symphony of weak bonds. Hydrogen bonds, ionic bonds, and hydrophobic interactions form a structural scaffold that maintains the enzyme’s precise shape. However, when these bonds succumb to external stressors, the protein chains start to unravel, like a tapestry torn apart thread by thread.
Disruption of Weak Bonds:
The first blow is struck when these weak bonds weaken or break. Temperature, pH changes, and chemical agents can exert their disruptive force, nudging the bonds toward their demise. As the bonds fail, the protein chains begin to lose their rigidity, like a once-firm pillar crumbling to dust.
Formation of Aggregates:
As the protein chains loosen their grip, hydrophobic groups that were once shielded within the enzyme’s core are now exposed to the aqueous environment. Like oil and water, hydrophobic groups naturally repel water, causing the unfolded chains to clump together, forming unsightly aggregates. These aggregates are like tangled knots, hindering the enzyme’s ability to interact with its substrates.
Net Charge Changes:
The unraveling of protein chains also exposes charged amino acid side chains. This disruption of the enzyme’s electrical balance results in net charge changes. These altered charges can further impact the enzyme’s solubility and its interactions with other molecules, exacerbating the denaturation process.
In the end, the enzyme’s once-precise structure is irrevocably compromised. The protein chains, once folded in a delicate dance, now lay in ruin, unable to perform the intricate choreography of catalysis. The enzyme, once a master of molecular transformations, is now reduced to a mere shadow of its former glory.
Aggregation of Unfolded Proteins: The Unraveling of Order
As the once-orderly protein chains unfold and unravel, they expose their hydrophobic regions to the aqueous environment. This exposure triggers a cascade of consequences that culminates in the formation of protein aggregates.
These aggregates, like tangled balls of thread, are less soluble than their native counterparts. As they grow in size, they sequester more hydrophobic groups, further driving the aggregation process. The increasing exposure of hydrophobic regions not only decreases solubility but also alters the net charge of the enzyme.
In its native state, an enzyme’s solubility is finely tuned by a delicate balance of hydrophilic and hydrophobic interactions. However, as hydrophobic groups are exposed, this balance is disrupted, leading to reduced solubility. Moreover, these changes in the enzyme’s charge distribution can affect its interactions with other molecules, including cofactors and substrates.
Loss of Enzyme Solubility: The Unraveling of Protein Structure
As enzyme denaturation unfolds, one of its most significant consequences is the loss of solubility. This phenomenon arises due to a direct correlation with changes in the enzyme’s net charge.
Imagine a protein as an intricately folded molecule held together by a symphony of weak bonds. Under normal conditions, these bonds maintain the enzyme’s native conformation, which ensures its solubility and functionality.
However, when denaturing forces such as heat or chemical agents disrupt these weak bonds, the protein’s structure begins to unravel. The unfolding protein chains expose hydrophobic groups that were previously buried within the molecule. These hydrophobic regions, which have an affinity for nonpolar environments, aggregate together, forming insoluble clusters.
Furthermore, the loss of solubility is also influenced by changes in the enzyme’s net charge. As the protein unfolds, charged amino acid side chains are exposed to the aqueous environment. These changes alter the enzyme’s overall charge, affecting its interactions with water molecules and other polar compounds, ultimately leading to a decrease in solubility.
The loss of solubility is a critical aspect of enzyme denaturation because it impairs the enzyme’s ability to interact with its substrate. Without sufficient solubility, the enzyme cannot effectively bind to its target, leading to a loss of catalytic activity.
In conclusion, the loss of enzyme solubility is a consequence of denaturation that is inextricably linked to changes in the enzyme’s net charge and unfolding of its protein chains. This loss of solubility has profound implications for enzyme function, as it hinders the enzyme’s ability to engage with its substrate, rendering it inactive.
Change in Net Charge: Impact on Enzyme Function and Solubility
Enzymes, the workhorses of our cells, are incredibly sensitive to their environment. A subtle shift in their molecular structure, known as denaturation, can disrupt their function and even lead to their demise. Net charge plays a pivotal role in this process, influencing both enzyme solubility and the stability of their interactions with vital cofactors.
As an enzyme undergoes denaturation, its protein chains begin to unravel, exposing previously hidden hydrophobic groups and altering its overall charge distribution. This change in charge affects the enzyme’s solubility. Positively charged enzymes, for example, become less soluble as denaturation exposes more negatively charged amino acids. The opposite occurs for negatively charged enzymes, which become more soluble with the exposure of positive charges.
Cofactors, small molecules that assist enzymes in their catalytic tasks, are often held in place by electrostatic interactions. The change in net charge during denaturation can disrupt these interactions, leading to cofactor release and a further loss of enzyme activity. This release of cofactors can further alter the charge distribution of the enzyme, creating a self-perpetuating cycle of denaturation.
The impact of net charge changes on enzyme function highlights the delicate balance of molecular interactions within these biological catalysts. Understanding these charge-related effects is crucial for researchers seeking to design enzymes with improved stability and activity, as well as for clinicians striving to diagnose and treat diseases that arise from enzyme dysfunction.
Exposure of Hydrophobic Groups
- Role in aggregation and net charge changes.
Exposure of Hydrophobic Groups
Within the intricate structure of enzymes, hydrophobic groups usually reside in a shielded environment away from water. However, when denaturation occurs, these hidden hydrophobic groups are exposed to the aqueous environment. This exposure plays a crucial role in both aggregation and net charge changes.
As hydrophobic groups come into contact with water, they strive to minimize their interaction with the polar solvent. This leads to the aggregation of unfolded proteins, forming dense clusters to reduce their exposure to water. This aggregation further stabilizes the denatured state and contributes to the overall loss of solubility.
Moreover, the exposure of hydrophobic groups can influence the net charge of the enzyme. Ionizable amino acid side chains, which are normally buried within the enzyme’s structure, become exposed to the solvent upon denaturation. This can lead to changes in the overall charge of the enzyme, affecting its solubility and interactions with other molecules.
In summary, the exposure of hydrophobic groups is a key event in the denaturation process. It promotes aggregation and net charge changes, ultimately contributing to the loss of solubility and loss of enzymatic activity.
Enzyme Denaturation
The Silent Enzyme Killer: A Deeper Dive into the Mechanisms of Enzyme Denaturation
What is Enzyme Denaturation?
Enzymes, the workhorses of our cells, are intricate protein molecules that catalyze essential biochemical reactions. However, these meticulous structures can fall prey to a process called denaturation, where they lose their functional shape and biological activity.
The Denaturing Assault
Denaturation arises from various factors, including heat, pH changes, and chemical agents, which disrupt the delicate bonds that maintain the enzyme’s structure. This triggers a cascade of events, beginning with the alteration of the enzyme’s shape.
Altered Enzyme Shape
As the enzyme unfolds, its protein chains lose their intricate folding patterns. This conformational change exposes hydrophobic groups that normally reside within the protein’s core. These exposed groups interact with the surrounding water molecules, leading to aggregation.
Unfolding Protein Chains and Aggregation
The unfolding of protein chains is driven by the disruption of weak bonds, such as hydrogen bonds and hydrophobic interactions. As a result, the unfolded proteins form aggregates, further exposing hydrophobic groups and reducing solubility.
Loss of Solubility and Charge Changes
The aggregation of unfolded proteins leads to a further decrease in solubility. In addition, the exposure of charged amino acid side chains on the protein’s surface alters its overall net charge.
Exposure of Hydrophobic Groups
The exposed hydrophobic groups not only contribute to aggregation but also influence the net charge of the enzyme. This charge change is crucial as it affects the enzyme’s solubility and ability to interact with other molecules.
Loss of Cofactors
Some enzymes require cofactors, small molecules essential for their activity. Denaturation can lead to the release of these cofactors, crippling the enzyme’s functionality. The loss of cofactors can also render the denaturation process irreversible.
Irreversibility of Denaturation
Denaturation is often an irreversible process. The extensive shape changes, protein unfolding, and aggregation that occur during denaturation make it incredibly challenging to restore the enzyme’s native conformation.
Understanding the mechanisms of enzyme denaturation not only provides valuable insights into the functioning of enzymes but also has practical implications in biotechnology and various industrial processes. By understanding the factors that contribute to enzyme denaturation, scientists can develop strategies to mitigate its effects and maintain enzyme activity in harsh environments.
Denaturation: The Silent Annihilation of Enzyme Activity
As we navigate the intricate realm of enzymes, we encounter a phenomenon known as denaturation, a process that subtly yet decisively alters their very essence. This silent transformation, if not understood, can lead to enzymatic catastrophe.
The Irreversibility of Denaturation
The cornerstone of denaturation’s destructive nature lies in its irreversibility. Once an enzyme succumbs to the forces of denaturation, its intricate structural scaffold unravels, rendering it incapable of resuming its catalytic duties. The once-precise molecular machinery responsible for orchestrating biochemical reactions is now a shadow of its former self.
This irreversibility stems from the profound conformational changes that accompany denaturation. The *enzyme’s shape, once perfectly tailored for its specific substrate, becomes distorted. Protein chains unfold, exposing previously concealed hydrophobic groups to the aqueous environment. These hydrophobic regions, like oil and water, repel the surrounding water molecules, leading to aggregation. Unfolded protein chains also disrupt weak bonds, further destabilizing the enzyme’s structure.
The formation of these aggregates further compounds the damage. They reduce the enzyme’s solubility, making it less likely to encounter its target substrate. The net charge of the enzyme also changes, affecting its solubility and interactions with cofactors. These cofactors, essential for enzyme activity, are often released during denaturation, leaving the enzyme crippled and unable to function.
Consequences of Enzyme Denaturation
The consequences of enzyme denaturation can be far-reaching. In living organisms, it can lead to a breakdown in cellular processes, disrupting metabolism and potentially causing disease. In industrial applications, it can compromise the efficiency and productivity of enzymes used in the production of food, pharmaceuticals, and biofuels.
Preventing Denaturation: A Quest for Enzyme Preservation
Understanding the mechanisms and consequences of denaturation is crucial for safeguarding enzymes and preserving their catalytic prowess. By controlling factors that can trigger denaturation, such as temperature, pH, and exposure to denaturing agents, we can ensure that enzymes remain in their native, functional state.
Denaturation stands as a formidable threat to enzyme activity, its irreversibility a constant reminder of the delicate nature of these biological catalysts. By unraveling the mechanisms behind this phenomenon, we gain insights into the preservation of enzyme function, ensuring that these molecular workhorses continue to drive the vital processes of life and industry.