Energy Removal From Matter: Techniques And Mechanisms
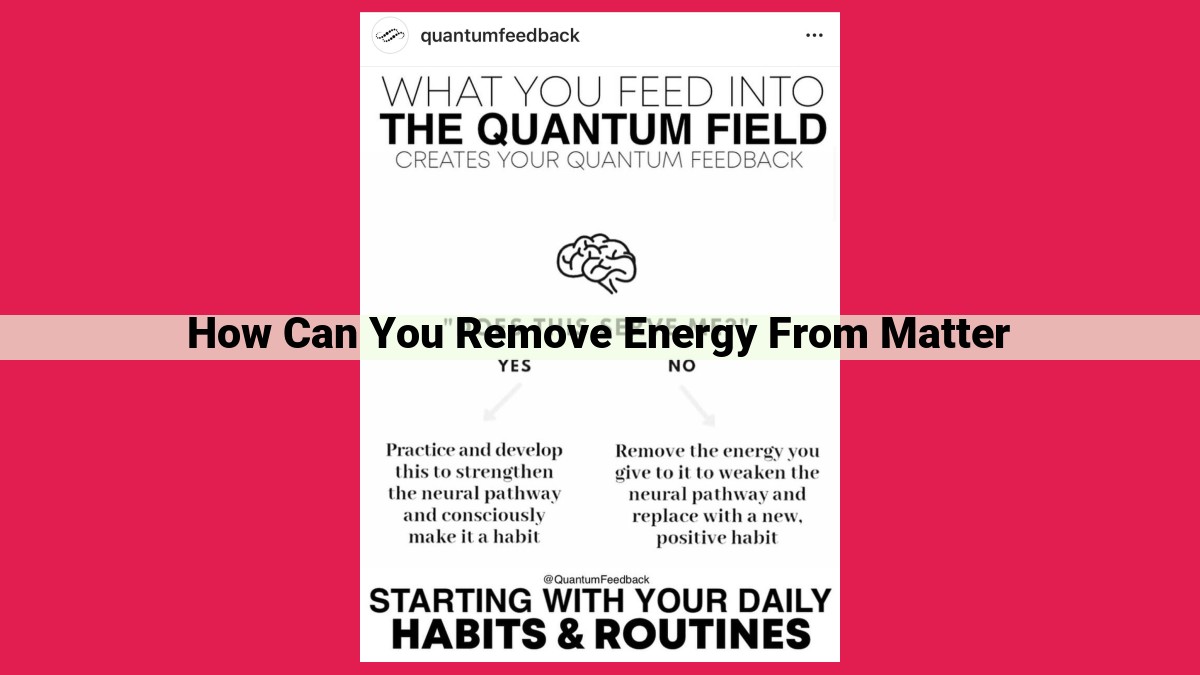
Energy removal from matter involves several methods: Heat transfer occurs when heat flows from hotter to colder objects, influenced by thermal conductivity, specific heat capacity, and heat flux. Work is performed when a force acts on an object over a distance, transferring energy. Matter change, including physical (e.g., melting) and chemical (e.g., burning) changes, alters the energy content of matter. Radiation, such as light or waves, can transfer energy through electromagnetic interactions. Electrical energy, characterized by voltage, current, resistance, and power, enables energy flow and transfer in electrical systems.
Heat Transfer: The Intricate Dance of Energy Movement
Thermal Conductivity: The Proficiency of Heat Conduction
Imagine a crowded dance floor where molecules are the eager dancers. Thermal conductivity measures how well these molecules pass along the energy, just like dancers sharing their moves. Substances with high thermal conductivity, like metals, get the party going quickly, allowing heat to flow easily through them. Poor conductors, like wood or plastic, stumble over each other, hindering the energy transfer.
Specific Heat Capacity: The Energy Storage Champs
Now let’s imagine each dancer has a secret stash of energy. Specific heat capacity measures how much energy it takes to raise the temperature of a substance by one unit. Water has a high specific heat capacity, acting like a “heat sponge” that absorbs and releases energy without大幅 increasing its temperature. This is why water is often used as a coolant.
Heat Flux: The Flow of Heat Energy
The final dance move is heat flux, representing the rate at which heat energy flows through a particular area. Imagine a stream of dancers rushing through a narrow passageway. The faster the dancers flow, the higher the heat flux. Materials with high thermal conductivity and a large temperature gradient (difference in heat) experience a more vigorous heat flux.
Energy and Work: A Dynamic Duo
Introduction:
In the tapestry of our physical world, energy and work are intertwined, forming an inseparable bond. Energy, the capacity to do work, manifests in various guises, driving all processes and transformations around us. Let’s delve into the captivating relationship between energy and work, exploring how it shapes our existence.
Energy and Work: Complementary Concepts
Imagine a solitary rock resting on a table. It possesses potential energy due to its position above the ground. When you apply force to push it off the table, the rock’s potential energy converts into kinetic energy, which is the energy of motion. As it falls, the rock’s kinetic energy increases, while its potential energy decreases. This simple demonstration illustrates the intimate connection between energy and work.
Work: A Force in Motion
Simply put, work is force applied over a distance. When you exert a force to move an object, you perform work. The amount of work done is determined by the magnitude of the force and the distance over which it is applied. In physics, work is measured in joules (J), representing the energy transferred or expended during the process.
Power: A Measure of Effortlessness
Power is the rate at which work is done. It measures how quickly you can perform work. A machine with high power can perform a significant amount of work in a given time, while a machine with low power will take longer to accomplish the same task. The formula for power is:
Power = Work / Time
In other words, power tells us how much work can be completed per unit time.
Conclusion:
In the vast panorama of our physical realm, energy and work orchestrate the symphony of change and motion. Energy enables work, while work transforms energy, driving natural phenomena and technological advancements. By understanding the interplay between these fundamental concepts, we gain a deeper appreciation for the intricate dance of the universe.
Matter Change: The Dance of Energy
Physical Changes:
Imagine a solid ice cube that you drop into a warm cup of water. Gradually, the ice cube begins to melt, transforming from a hard, crystalline structure into a liquid. This process of melting, triggered by an increase in temperature, is an example of a physical change. Physical changes do not alter the molecular structure of the substance. Instead, they change its physical properties, such as shape, volume, or density.
Chemical Changes:
Unlike physical changes, chemical changes involve the rearrangement of atoms and the formation of new substances. Think of burning paper: the paper changes from a solid to an ash, releasing heat and light. This is because the cellulose molecules in the paper react with oxygen to form carbon dioxide and water vapor. Chemical changes often involve exothermic reactions (releasing energy) or endothermic reactions (absorbing energy).
Energy in Matter Transformation
Matter changes can significantly affect the energy content of the substance. Physical changes generally require less energy than chemical changes. Melting and freezing involve changes in energy as molecules gain or lose kinetic energy. Chemical changes often release or absorb large amounts of energy due to the formation or breaking of chemical bonds.
States of Matter
The three fundamental states of matter are solid, liquid, and gas. Solids have a definite shape and volume, while liquids have a definite volume but no definite shape. Gases neither have a definite shape nor a definite volume. These states are determined by the energy level of the molecules. Solids have the lowest energy level, followed by liquids and then gases. As energy increases, molecules gain kinetic energy, allowing them to move more freely, leading to a change in state.
Radiation: The Invisible Force of Energy
Radiation, an enigmatic yet ubiquitous force, permeates our universe, carrying energy across vast distances. It manifests in a spectrum of forms, from the gentle warmth of sunlight to the insidious power of X-rays.
The Electromagnetic Spectrum: A Symphony of Waves
Radiation is an integral part of the electromagnetic spectrum, a continuous range of waves characterized by their wavelength (distance between peaks) and frequency (number of peaks per second). The spectrum encompasses a vast array of visible light, as well as invisible forms such as infrared, ultraviolet, gamma, and radio waves.
Light and Waves: The Dual Nature of Radiation
Light, a visible form of radiation, exhibits both particle-like and wave-like properties. As particles, called photons, light carries quantized units of energy. Simultaneously, it behaves as a wave, characterized by its speed of propagation, wavelength, and interference patterns.
Other forms of radiation, such as radio waves, also possess wave-like nature, allowing them to transmit information and penetrate materials. The frequency of radiation determines its ability to interact with different substances and penetrate matter. Higher-frequency radiation, such as X-rays and gamma rays, can penetrate deeply, while lower-frequency radiation, such as radio waves, interacts more with superficial layers.
Through its ability to traverse vast distances and interact with matter, radiation plays a pivotal role in our understanding of the universe and its impact on life on Earth.
Electrical Energy: The Invisible Force that Powers Our World
Voltage: The Driving Force
Imagine electricity as a river flowing through wires. The voltage is analogous to the height of a waterfall, determining the potential energy of the electrons passing through. A higher voltage creates a stronger driving force, pushing electrons with greater energy.
Current: The Flow of Electrons
The current represents the rate at which electrons move through the conductor. Think of it as the volume of water flowing through a pipe. A stronger current indicates a greater flow of electrons, transporting more energy.
Resistance: The Impeding Force
As electrons travel through wires, they encounter resistance, similar to friction in a flowing river. Resistance is measured in ohms and impedes the flow of electrons, converting some of their energy into heat. The higher the resistance, the more difficult it becomes for electrons to move.
Power: The Ultimate Measure
Power is the rate at which electrical energy is transferred. It is calculated by multiplying the voltage by the current, analogous to the product of a waterfall’s height and the volume of water flowing. Electrical devices consume power, converting it into other forms of energy, such as heat, light, or motion.
The Dance of Electrons
Understanding these factors is crucial for harnessing electrical energy effectively. When voltage, current, resistance, and power are balanced, electrical devices function seamlessly. However, imbalances can lead to inefficiencies, overheating, or even damage to components.
Electrical energy, with its intricate interplay of voltage, current, resistance, and power, is a fundamental force that plays a vital role in our modern world. By understanding these concepts, we can unlock the immense potential of electricity and harness its power to shape our lives.