Energy Flow In Ecosystems: Understanding Photosynthesis, Cellular Respiration, And Food Chains
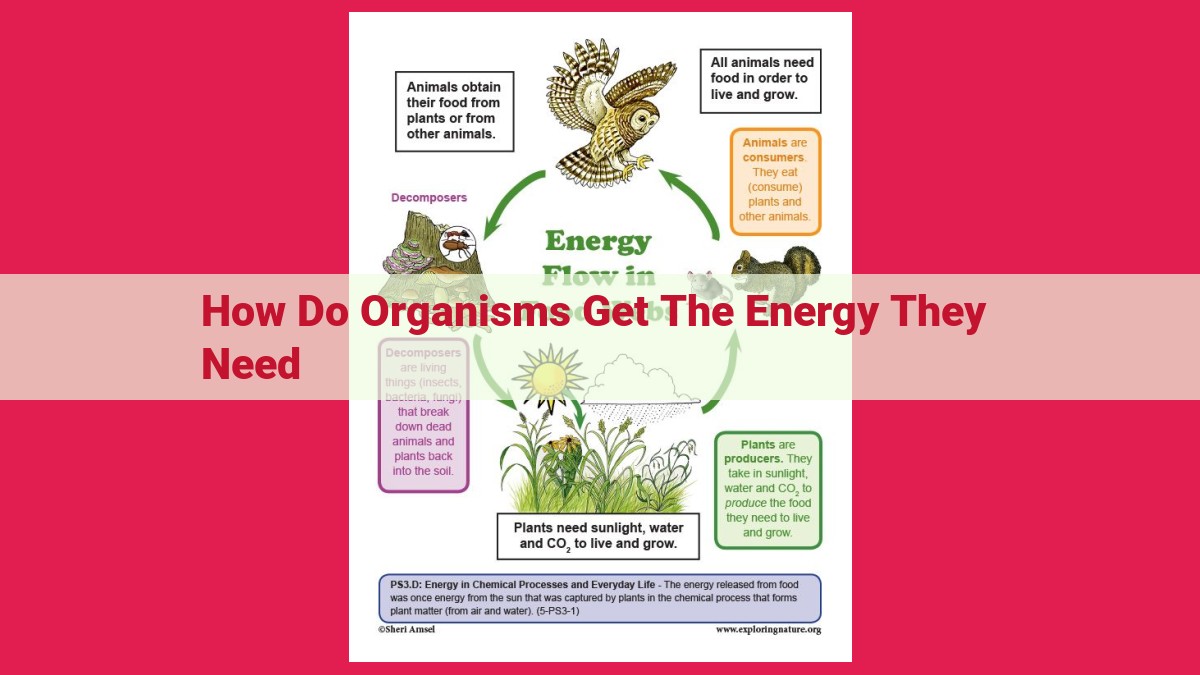
Organisms acquire energy through two primary pathways: photosynthesis and cellular respiration. Photosynthesis utilizes sunlight to convert carbon dioxide into sugars, while cellular respiration breaks down organic molecules to produce ATP, the cell’s energy currency. Autotrophs, such as plants, use photosynthesis to synthesize food; heterotrophs, including animals, consume autotrophs to obtain energy. The energy flow through ecosystems is represented by food chains and food webs, demonstrating the transfer of energy from producers to consumers.
Energy Acquisition in Organisms: A Vital Life Process
At the heart of all biological processes lies energy acquisition, a fundamental cornerstone of life. From the tiniest organisms to the grandest ecosystems, living entities rely on a steady supply of energy to sustain their existence.
Photosynthesis: Harnessing the Sun’s Rays
Some organisms possess the remarkable ability to turn sunlight into chemical energy through the process of photosynthesis. Chlorophyll-containing cells, such as those found in plants and algae, act as solar panels, capturing light energy and using it to convert carbon dioxide and water into glucose, a high-energy sugar molecule.
Cellular Respiration: Fueling the Body
Other organisms rely on cellular respiration to generate energy. This complex process occurs within the cells’ mitochondria and involves breaking down glucose, releasing the stored energy in a stepwise manner.
Glycolysis: The Initial Step
Glycolysis initiates cellular respiration, breaking down glucose into smaller molecules and producing a small amount of ATP, the cell’s energy currency. The end products of glycolysis, pyruvate and NADH, feed into subsequent stages of respiration.
Krebs Cycle: Energy Powerhouse
The Krebs cycle, also known as the citric acid cycle, occupies center stage in cellular respiration. Here, pyruvate undergoes a series of reactions, releasing carbon dioxide and generating much ATP, NADH, and FADH2.
Electron Transport Chain: Proton Pumping Factory
The electron transport chain harnesses the energy stored in NADH and FADH2 to create a proton gradient across the mitochondrial membrane. This gradient drives a proton pump, which ultimately generates large amounts of ATP.
Fermentation: An Alternative Pathway
In the absence of oxygen, some organisms resort to fermentation. This process allows them to extract energy from glucose without the need for cellular respiration. Fermentation produces various end products, including lactic acid in muscle cells and ethyl alcohol in yeast.
Photosynthesis: Nature’s Solar-Powered Energy Factory
What is Photosynthesis?
Photosynthesis is the fundamental process by which plants and certain other organisms harness the energy of sunlight to produce their own nourishment. It’s the backbone of life on Earth, as it sustains not only plants but also the animals and other organisms that depend on them for food.
The Actors in Photosynthesis
The key players in photosynthesis are chlorophyll, a green pigment found in chloroplasts, the energy-converting organelles within plant cells. Chlorophyll has an amazing ability to trap light energy from the sun.
Light Energy Conversion
When sunlight strikes chlorophyll, photons of light energy are absorbed and used to split water molecules into hydrogen and oxygen. The oxygen is released as a byproduct, while the hydrogen is combined with carbon dioxide to produce glucose, a sugar molecule that serves as the plant’s primary energy source.
The Importance of Photosynthesis
Photosynthesis is an indispensable process for life on our planet. It provides the oxygen we breathe and the food we eat. It also plays a crucial role in regulating the Earth’s climate by absorbing carbon dioxide from the atmosphere.
Photosynthesis is a remarkable natural phenomenon that sustains the delicate balance of life on Earth. Its ability to harness the energy of sunlight and convert it into food is a testament to the ingenuity and complexity of the natural world.
Cellular Respiration: Fueling Life’s Processes
In the symphony of life, every biological process requires energy. One such process, essential for all living organisms, is cellular respiration. Picture this cellular dance as a symphony, where molecules interact in a sequence of events to produce the energy currency of life: ATP.
Cellular respiration, happening right within our cells, is akin to the powerhouse that fuels our biological machinery. It’s a multi-step process akin to a relay race, with each step playing a vital role. Let’s dive into this metabolic saga.
Glycolysis, the first stage, acts like the starting line, where glucose, the cellular energy source, is broken down into simpler compounds. Next, the Krebs cycle, also known as the citric acid cycle, takes the baton, oxidizing these compounds further. This dance of oxidation releases carbon dioxide as a byproduct and generates high-energy electron carriers.
The electron transport chain, the final stage, is the grand finale, where those energetic electrons are passed down a series of molecules, creating a proton gradient across the mitochondrial membrane. This gradient powers the synthesis of ATP through a molecular machine known as ATP synthase. It’s like a spinning turbine, constantly replenishing the cell’s energy supply.
Cellular respiration, like a well-coordinated symphony, ensures a continuous flow of energy to sustain life’s processes. From muscle contractions to nerve impulses, every cellular function relies on this intricate dance of energy production.
Glycolysis: The Gateway to Energy Production
In the realm of cellular energy acquisition, glycolysis emerges as the gateway to a cascade of biochemical reactions essential for life. This critical process initiates the breakdown of glucose, the body’s primary fuel source, embarking on a journey that culminates in the generation of ATP, the cellular currency of energy.
The Krebs cycle, a subsequent stage in cellular respiration, relies heavily on the products of glycolysis to continue the energy production process. Pyruvate, a key end product of glycolysis, serves as the starting molecule for the Krebs cycle. Through a series of intricate chemical transformations, acetyl-CoA, a molecule rich in energy potential, is produced and fed into the Krebs cycle to complete the energy-yielding pathway.
Beyond its direct involvement in energy generation, glycolysis also plays a pivotal role in cellular homeostasis. By regulating glucose utilization and producing intermediary metabolites, it supports a diverse range of cellular functions, from nutrient sensing to cell signaling.
In essence, glycolysis stands as a fundamental cornerstone in the intricate dance of cellular energy acquisition. Its significance extends far beyond its isolated role, as it sets the stage for subsequent metabolic pathways, enabling the cell to flourish and perform its myriad functions.
The Krebs Cycle: Powerhouse of Cellular Respiration
At the heart of cellular energy production lies a remarkable biochemical pathway known as the Krebs cycle. This intricate dance of chemical reactions is responsible for generating a significant portion of the ATP (adenosine triphosphate) that powers our biological processes.
The Krebs cycle, also known as the citric acid cycle, takes place within the matrix of mitochondria, the energy powerhouses of cells. It begins with a molecule of acetyl-CoA, which is derived from the breakdown of carbohydrates, fats, and proteins during glycolysis.
As acetyl-CoA enters the cycle, it combines with oxaloacetate to form citrate. Through a series of enzymatic reactions, citrate undergoes a series of transformations, releasing carbon dioxide, high-energy electrons, and ATP.
The high-energy electrons are captured by NADH and FADH2, which carry them to the electron transport chain, where they will ultimately be used to generate more ATP. ATP is the universal energy currency of cells, providing the power for cellular functions such as muscle contraction, protein synthesis, and nerve impulse transmission.
The Krebs cycle also plays a crucial role in the breakdown of glucose, the body’s primary energy source. Each glucose molecule generates two acetyl-CoA molecules, which then enter the Krebs cycle twice, releasing a total of six molecules of carbon dioxide and generating a significant amount of ATP.
In summary, the Krebs cycle is a vital metabolic pathway that serves as the primary source of ATP production in cells. This cycle transforms acetyl-CoA into carbon dioxide and generates high-energy electrons, which are used to generate even more ATP through the electron transport chain. Without the Krebs cycle, our cells would quickly run out of energy, and life as we know it would cease to exist.
Electron Transport Chain: Generating the Energy Currency
- Discuss the location and structure of the electron transport chain, explaining how it establishes a proton gradient for ATP production.
The Electron Transport Chain: Generating the Energy Currency
In the symphony of cellular respiration, the electron transport chain emerges as the grand finale, the culmination of a series of choreographed steps that transform the stored energy in glucose into the universal energy currency: ATP. Nestled within the folds of the inner mitochondrial membrane, the electron transport chain is a molecular assembly of immense complexity and sophistication.
At its core lies a series of multi-protein complexes, each designed to pass electrons from one molecule to the next, like a relay race with oxygen as the eager baton recipient. Electrons, derived from the breakdown of glucose during glycolysis and the Krebs cycle, embark on this journey through the chain. As they pass through each complex, their energy is harnessed to create a proton gradient across the mitochondrial membrane.
Think of this proton gradient as a miniature dam, storing the potential for energy release. Protons, positively charged particles, accumulate on one side of the membrane, creating a separation of charge. This electrochemical gradient provides the driving force for ATP synthase, a turbine-like enzyme embedded in the membrane.
As protons rush through ATP synthase, driven by the gradient, they spin the enzyme, causing a conformational change that synthesizes ATP. ATP, the energy currency of cells, is then used to power countless cellular processes, from muscle contraction to nerve transmission.
The electron transport chain is not merely an isolated entity but an integral part of the cellular respiration orchestra. Its ability to generate ATP is fueled by the high-energy electrons derived from the breakdown of glucose. In turn, the protons pumped across the membrane by the electron transport chain provide the energy for ATP synthase to generate the energy currency that drives life’s essential processes.
In essence, the electron transport chain is the conductor of cellular respiration, orchestrating the flow of electrons and protons to produce ATP, the energy that powers the symphony of life.
Fermentation: An Alternative Source of Energy
When the primary energy-generating processes like photosynthesis and cellular respiration are compromised or insufficient, organisms resort to fermentation as a backup energy-generating process.
Types, End Products, and Significance of Fermentation
Fermentation occurs in the absence of oxygen and typically involves the breakdown of organic molecules, such as glucose, to produce energy-rich molecules and other byproducts.
There are two main types of fermentation:
-
Lactic acid fermentation, common in bacteria and some animal cells, produces lactic acid as the main byproduct. This process is utilized in the production of yogurt, cheese, and sauerkraut.
-
Alcoholic fermentation, found in yeast and some bacteria, converts glucose into ethanol (alcohol) and carbon dioxide. This process is essential in brewing, winemaking, and baking.
Fermentation: A Critical Survival Mechanism
Fermentation is a critical survival mechanism for organisms in low-oxygen environments or when cellular respiration is impaired. For example, muscle cells can switch to lactic acid fermentation during intense exercise when oxygen supply is limited.
Despite its importance, fermentation produces less energy compared to cellular respiration and can lead to the accumulation of waste products, which can have negative consequences on cell function if not properly managed.
In conclusion, fermentation is an alternative energy pathway that allows organisms to generate energy in the absence of oxygen. Its various forms and end products have significant implications in food production and metabolic processes. Understanding fermentation provides valuable insights into cellular metabolism and the adaptations organisms employ to survive in diverse environmental conditions.
Autotrophs: The Green Powerhouses of Life
In the grand symphony of life, organisms play diverse roles, but none is as crucial as that of autotrophs, the primary producers. Autotrophs are the life forms capable of synthesizing their own food using inorganic compounds and an external energy source. This extraordinary ability makes them the foundation of the entire food chain and the ultimate source of energy in our ecosystems.
The most well-known autotrophs are plants, which use sunlight to drive the process of photosynthesis. This remarkable reaction captures the sun’s energy and converts it into chemical energy, stored within glucose molecules. As they create their own sustenance, plants simultaneously release oxygen into the atmosphere, a vital byproduct for all aerobic organisms.
But autotrophs extend beyond the vibrant greenery of plants. Some bacteria and algae also possess the photosynthetic machinery, enabling them to harness sunlight’s power. These microorganisms thrive in diverse environments, including oceans, lakes, and even extreme geothermal springs.
The significance of autotrophs cannot be overstated. They are the primary producers, converting raw materials into organic matter that fuels all other life on Earth. Without them, the intricate web of life would collapse, leaving us in the dark without the energy to thrive.
Heterotrophs: The Consumers in the Energy Flow
In the intricate web of life, the heterotrophs stand as the consumers, playing a crucial role in the continuous cycle of energy flow. Unlike autotrophs (primary producers) that harness sunlight or chemical energy to synthesize their own food, heterotrophs are dependent on external sources of energy and nutrients.
Heterotrophs include a vast array of organisms, from the tiniest bacteria to the mighty whales. These creatures cannot synthesize their own complex organic molecules and therefore must consume other organisms to obtain the necessary energy and nutrients for survival.
Heterotrophs play a pivotal role in the ecosystem by breaking down complex organic matter into simpler substances that can be utilized by other organisms. This process, known as decomposition, is essential for nutrient cycling and maintains the overall balance of ecosystems.
As heterotrophs consume organisms, they pass energy and nutrients up the food chain, ultimately supporting the entire food web. Food chains represent a linear sequence of energy transfer from primary producers to top predators. Each level of the food chain, known as a trophic level, represents a transfer of about 10% of the energy consumed by the previous level.
Food webs are more complex representations of energy flow within ecosystems, acknowledging the interconnectedness of food chains. In food webs, multiple food chains overlap, creating a network of energy exchange. This complexity helps maintain ecosystem stability, resilience, and nutrient cycling.
Heterotrophs can be further classified based on their feeding habits:
- Herbivores consume plants and algae.
- Carnivores consume animals.
- Omnivores consume both plants and animals.
- Detritivores consume decaying organic matter.
- Parasites obtain nutrients from living hosts without killing them.
Each type of heterotroph plays a unique role in the ecosystem, contributing to the overall balance and stability of life on Earth.
Food Chain: The Linear Flow of Energy
As we delve into the intricacies of energy acquisition in organisms, we encounter the concept of food chains. A food chain is a linear sequence of organisms through which energy and nutrients flow, from the initial producer to the ultimate consumer.
At the foundation of every food chain lies the primary producer, typically an autotrophic organism such as a plant or algae. These organisms harness the power of sunlight through photosynthesis to convert carbon dioxide and water into glucose and oxygen. This glucose, the primary source of energy for all life, is then passed along the food chain.
Next in line are the primary consumers, herbivores that feed directly on the primary producers. They convert the energy stored in plants into their body tissues. These consumers, in turn, become sustenance for secondary consumers, carnivores that prey on them. This process of energy transfer continues through multiple trophic levels, each succeeding level representing a higher consumer.
Along the food chain, energy is lost at each trophic level due to metabolism and other physiological processes. As a result, the biomass available at each level decreases as we move up the chain. This energy loss ultimately limits the length of food chains, typically ranging from three to five trophic levels in most ecosystems.
Understanding food chains is crucial for comprehending how energy flows through an ecosystem and how organisms interact. It also highlights the importance of biodiversity in ensuring the stability and resilience of these intricate networks of life.
Food Web: The Interconnected Network of Energy
Imagine a bustling metropolis, where individuals play diverse roles and contribute to the overall functioning of the city. In the realm of ecology, food webs are analogous to these urban landscapes, teeming with organisms interconnected through a intricate network of energy flow.
Food webs extend the concept of food chains, which depict linear energy pathways from producers to consumers. In a food web, multiple food chains overlap and intersect, forming a complex web of interactions. This intricate tapestry is vital for maintaining ecosystem dynamics and ensuring the cycling of nutrients.
At the foundation of food webs lie autotrophs, the primary producers that harness energy from the sun or inorganic molecules to create organic matter. These organisms, including plants, algae, and some bacteria, are the engines of energy capture, using chlorophyll or other pigments to convert light energy into chemical energy.
Grazing upon autotrophs are herbivores, the primary consumers. These animals, such as deer, rabbits, and insects, feed directly on plant matter, converting the plant’s stored energy into their own. Subsequent trophic levels include carnivores, animals that consume herbivores, and omnivores, organisms with a mixed diet ranging from plants to animals.
The interconnectedness of food webs becomes apparent at higher trophic levels. Predators often target multiple prey species, and prey species may have several predators. This web-like structure ensures that no single species dominates the ecosystem, maintaining a delicate balance and preventing overpopulation.
The intricate connections within food webs facilitate the cycling of nutrients. As organisms consume and digest their food, nutrients are released back into the environment. These nutrients, such as carbon, nitrogen, and phosphorus, are then available for uptake by autotrophs, completing the cycle.
Food webs play a crucial role in shaping ecosystem dynamics. The abundance and diversity of species, as well as the overall stability of the ecosystem, are influenced by the complexity and interconnectedness of the food web. Understanding these intricate relationships is essential for conservation efforts, ensuring the preservation of biodiversity and the resilience of ecological communities.