Understanding Element Reactivity: Factors And Their Impact
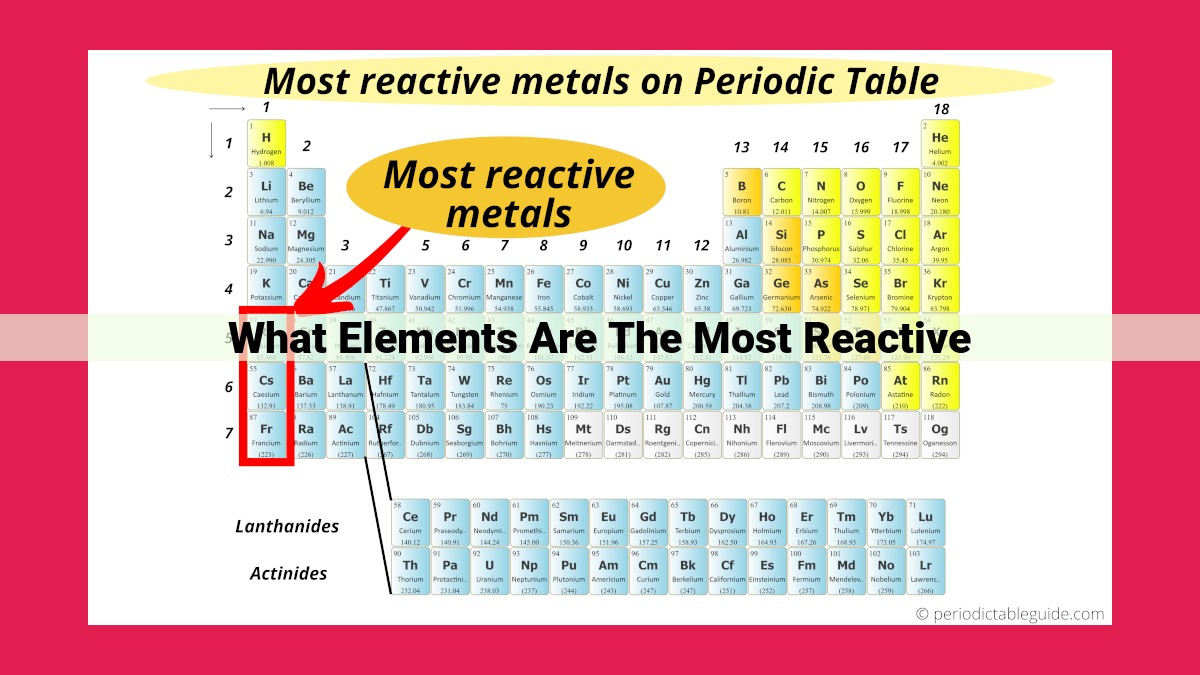
Reactivity refers to an element’s tendency to undergo chemical reactions. Factors influencing reactivity include:
- Ionization energy, the energy required to remove an electron, which decreases with increasing atomic size and valence electrons (more reactive).
- Electron affinity, the energy change when an atom gains an electron, which increases with decreasing atomic size and increasing valence electrons (more reactive).
- Atomic radius, the distance from the nucleus to the outermost electron shell, which increases down a group and decreases across a period (larger radii are more reactive).
- Electronegativity, the ability of an atom to attract electrons, which increases across a period and decreases down a group (more electronegative elements are more reactive).
Understanding Chemical Reactivity: The Essence of Transformations
What is Reactivity?
Reactivity is a fundamental concept in chemistry that describes the tendency of a substance to undergo chemical transformations. It determines the ease with which a substance reacts with other elements or compounds to form new substances. The importance of reactivity cannot be overstated, as it governs the formation of everything from life-sustaining molecules to the synthetic materials that shape our modern world.
Factors Influencing Reactivity
Several factors influence the reactivity of a substance. One crucial aspect is chemical bonding. The type of chemical bonds formed between atoms determines the stability of the molecule and, therefore, its resistance to change. Substances with weaker bonds are generally more reactive as they have a lower energy barrier to rearrange their atoms into new configurations.
Another factor that impacts reactivity is reaction rates. The rate at which a substance reacts can vary significantly depending on factors such as temperature, concentration, and the presence of catalysts. Higher temperatures and concentrations typically lead to faster reaction rates, while the presence of catalysts can accelerate reactions by providing an alternative pathway with a lower activation energy requirement.
Ionization Energy: A Key Player in Chemical Reactivity
In the realm of chemistry, reactions are the lifeblood of transformations. And at the heart of every reaction lies a fundamental property: ionization energy.
Ionization energy is the energy required to remove an electron from an atom or ion. It’s a crucial factor in determining the reactivity of an element. Elements with low ionization energies are more likely to lose electrons and become positively charged ions. Conversely, elements with high ionization energies hold onto their electrons more tightly, making them less reactive.
The size of an atom has a significant impact on its ionization energy. Larger atoms have their electrons spread out over a wider volume, resulting in lower ionization energies. This is because the electron is farther away from the nucleus and therefore experiences less attractive force.
Valence electrons, the electrons in the outermost energy level, also play a crucial role. Elements with more valence electrons tend to have lower ionization energies because these electrons are more loosely bound to the atom.
Ionization energy is not only a measure of reactivity but also has implications for chemical bonding. Elements with low ionization energies are more prone to forming ionic bonds, where one atom transfers an electron to another. On the other hand, elements with high ionization energies are more likely to form covalent bonds, where atoms share electrons.
In summary, ionization energy is a fundamental property that influences the reactivity of elements, the type of chemical bonds they form, and ultimately the outcome of chemical reactions. Understanding ionization energy enables chemists to predict and manipulate the behavior of elements, paving the way for a myriad of scientific and technological advancements.
Electron Affinity
- Definition and connection to reactivity
- Impact of atomic size and valence electrons on electron affinity
Electron Affinity: A Key Player in Chemical Reactivity
In the realm of chemistry, understanding the properties of atoms is crucial for deciphering their behavior in reactions. One such property that holds immense significance in determining reactivity is electron affinity.
Definition and Reactivity Connection
Electron affinity refers to the energy released when an atom accepts an electron. The higher the electron affinity of an element, the stronger its attraction for electrons. This property plays a pivotal role in chemical bonding, as atoms with high electron affinities tend to form ionic bonds by accepting electrons from other atoms. Conversely, elements with low electron affinities are more likely to donate electrons in covalent bonds.
Impact of Atomic Size and Valence Electrons
The atomic size of an element significantly influences its electron affinity. Larger atoms have a weaker attraction for electrons due to the increased distance between the nucleus and the outermost electrons. Hence, larger elements tend to have lower electron affinities.
On the other hand, the number of valence electrons also plays a role. Valence electrons are the outermost electrons in an atom, and their presence affects the electron affinity of an element. Elements with more valence electrons generally have higher electron affinities because these electrons experience a stronger attraction to the nucleus due to reduced electron-electron repulsion.
Electron affinity is an essential property for understanding the chemical behavior of elements. Its influence on reactivity, through the formation of ionic and covalent bonds, underscores its importance in predicting the properties and interactions of matter. By comprehending the intricate relationship between electron affinity and atomic size and valence electrons, we gain invaluable insights into the fundamental building blocks of the chemical world.
Atomic Radius
- Types of atomic radii and their significance: ionic radius, covalent radius
- Relationship between atomic radius and bonding properties
Atomic Radius: Unraveling the Size and Bonding Prowess of Atoms
The concept of atomic radius refers to the distance from the nucleus to the outermost electron shell of an atom. This seemingly simple measurement holds profound implications for an atom’s behavior and its ability to form chemical bonds.
There are two main types of atomic radii:
- Ionic Radius: This measures the size of an atom when it has lost or gained electrons, forming an ion.
- Covalent Radius: This represents the size of an atom when it forms a covalent bond, sharing electrons with another atom.
The atomic radius plays a crucial role in determining the bonding properties of atoms:
- Smaller atoms tend to have higher ionization energies but lower electron affinities. This means they resist losing electrons but readily accept them, exhibiting a higher tendency to form ionic bonds.
- Larger atoms have the opposite behavior. They are less likely to lose electrons and more likely to gain them, favoring the formation of covalent bonds.
The periodic trends in atomic radius are also noteworthy. As you move down a group (column) of the periodic table, the atomic radius generally increases due to the addition of new energy levels. Moving across a period (row), the atomic radius typically decreases due to the increased nuclear charge pulling the electrons closer to the nucleus.
Understanding atomic radius enables chemists to predict the bonding behavior and reactivity of elements. For example, the small size of fluorine makes it highly electronegative, leading to its strong ability to accept electrons and form ionic bonds with metals. In contrast, the large size of iodine contributes to its low reactivity, making it more likely to form covalent bonds.
In essence, atomic radius serves as a key factor in shaping the chemical landscape. By understanding the size of atoms and their bonding preferences, scientists can decipher the interactions between elements and predict the properties of countless compounds.
Electronegativity: The Invisible Force Shaping Chemical Bonds
In the realm of chemistry, the attraction between atoms can be quantified by a fundamental property known as electronegativity. Simply put, electronegativity measures an atom’s ability to attract electrons towards itself. This invisible force plays a crucial role in determining the type and strength of chemical bonds formed between atoms.
Electronegativity is not a random attribute but is closely related to an atom’s ionization energy and electron affinity. Ionization energy represents the energy required to remove an electron from an atom, while electron affinity measures the energy released when an electron is added. An atom with a high electronegativity generally has a high ionization energy, indicating it resists losing electrons, and a high electron affinity, suggesting it strongly attracts electrons.
The periodic table reveals a fascinating pattern in electronegativity. As we move from left to right across a row (period), electronegativity increases. This trend arises from the increasing number of protons in the nucleus, which strengthens the electrostatic attraction for electrons. Conversely, electronegativity decreases as we move down a column (group) in the periodic table. The addition of energy levels weakens the nucleus’s hold on electrons.
Understanding electronegativity is essential for comprehending the formation of chemical bonds. Atoms with similar electronegativities tend to form nonpolar covalent bonds where electrons are shared equally between them. When atoms with dissimilar electronegativities interact, polar covalent bonds form, with electrons being pulled towards the more electronegative atom. The difference in electronegativity between atoms determines the polarity of a bond.
In summary, electronegativity is an atomic property that governs the attraction of electrons and influences the formation of chemical bonds. By understanding electronegativity, chemists can predict the polarity of bonds and the behavior of molecules in chemical reactions.
Periodic Trends: Unraveling the Periodic Table’s Secrets
In the realm of chemistry, patterns and trends abound, guiding our understanding of the elements and their interactions. One such tapestry of patterns is known as periodic trends, which emerge from the organization of elements in the periodic table.
These trends are intimately connected to the atomic structure of elements. As we move across a row (period) or down a column (group) in the periodic table, the number of electrons, protons, and atomic orbitals changes. This symphony of changes orchestrates the properties of elements.
Ionization energy, the energy required to remove an electron from an atom, increases from left to right across a period. This is because the increase in the number of electrons in the outer shell creates a stronger attraction between the nucleus and the electrons. Conversely, down a group, ionization energy decreases due to the increasing distance between the nucleus and the outermost electrons.
Complementing ionization energy is electron affinity, the energy released when an electron is added to an atom. This trend follows a similar pattern, increasing from right to left across a period and decreasing down a group. As the number of orbitals increases down a group, it becomes easier for electrons to enter the outermost shell.
The atomic radius of an element, a measure of the size of its electron cloud, is inversely related to ionization energy and electron affinity. Across a period, atomic radius decreases from left to right as the increasing nuclear charge pulls electrons closer to the nucleus. Down a group, atomic radius increases due to the addition of new energy levels and the shielding effect of inner electrons.
Finally, electronegativity, the ability of an atom to attract electrons in a bond, increases from left to right across a period and decreases down a group. This trend mirrors ionization energy as it reflects the ease with which an atom can release or gain electrons.
Together, these periodic trends provide a roadmap for predicting the chemical behavior and reactivity of elements. From the formation of ionic bonds to the stability of covalent compounds, the periodic table serves as a guidebook for understanding the complexities of chemical interactions.
As we delve deeper into the periodic table, we unravel the secrets of chemistry, one element at a time. The tapestry of periodic trends empowers us to make sense of the chemical world and explore new frontiers of scientific discovery.