Understanding Factors Affecting Element Reactivity: Atomic Properties And Chemical Behavior
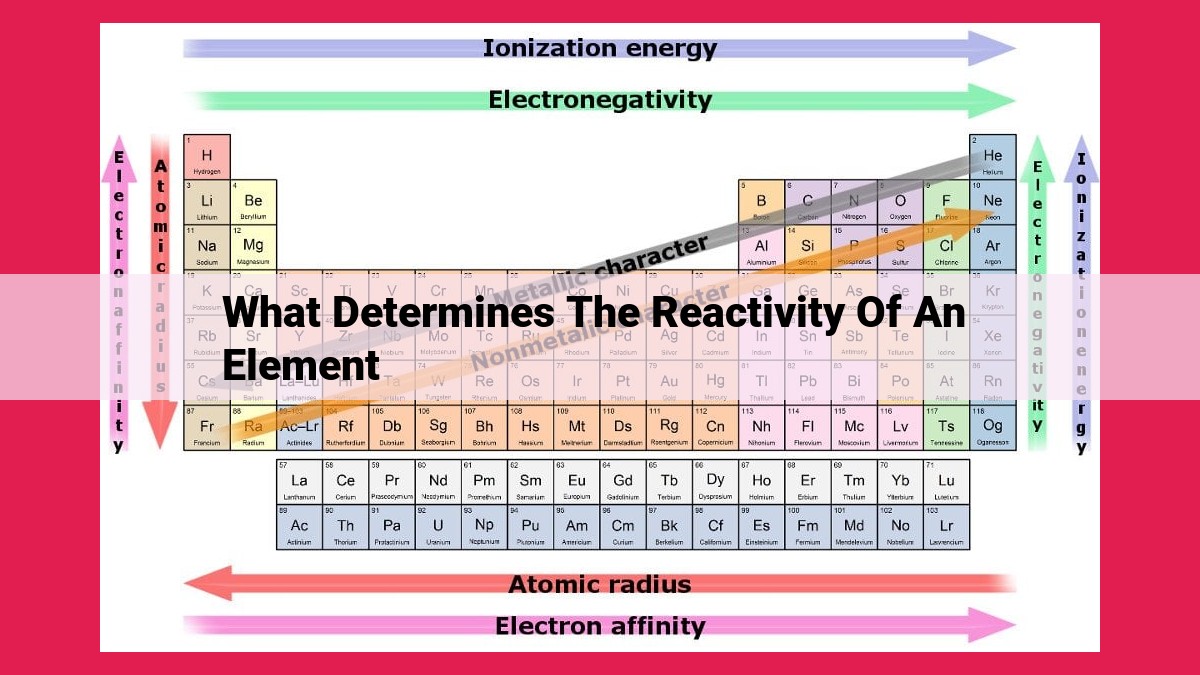
The reactivity of an element is significantly influenced by its atomic structure and properties. Factors such as atomic radius, ionization energy, electron affinity, electronegativity, valence electrons, metallic character, and non-metallic character play crucial roles in determining how reactive an element is. These properties are interconnected and provide insights into an element’s tendency to participate in chemical reactions, lose or gain electrons, and form stable compounds. Understanding these factors is essential for comprehending the behavior and reactivity of elements in various chemical contexts.
Atomic Structure and Properties
- Explain the concept of atomic radius, nuclear radius, and electron cloud.
Atomic Structure and Properties: Unveiling the Building Blocks of Chemistry
In the realm of chemistry, understanding the fundamental structure of atoms is pivotal to grasping the behavior and reactivity of elements. Let’s embark on a journey of atomic exploration, delving into the concepts of atomic radius, nuclear radius, and electron cloud, which are crucial for deciphering the properties of matter.
Atomic Radius: The Size of the Atomic Core
Imagine the atom as a miniature solar system, with the nucleus at its heart. The atomic radius represents the distance from the nucleus to the outermost boundary of an atom’s electron cloud. It reflects the number of electrons in the atom, increasing as you move down elemental groups from top to bottom.
Nuclear Radius: The Dense Heart of Matter
At the core of the atom lies the nucleus. This tiny, incredibly dense sphere contains positively charged protons and neutral neutrons. The nuclear radius is the measure of the nucleus’s size, and it is much smaller than the atomic radius. The nucleus holds the atom together, providing the gravitational force that counteracts the electrostatic repulsion between electrons.
Electron Cloud: The Mysterious Orbitals
Surrounding the nucleus is a vast, diffuse region called the electron cloud. It is where electrons reside, but not as tiny, point-like particles. Instead, electrons occupy orbitals, regions of space where the probability of finding an electron is highest. The electron cloud is dynamic and constantly shifting, creating a complex three-dimensional distribution of electron density.
Ionization and Electron Affinity: Unveiling the Atomic Dance
In the enigmatic world of atoms, electrons dance around their nucleus, governed by the laws of quantum mechanics. Two fascinating properties that shed light on this intricate dance are ionization energy and electron affinity. Let’s delve into these concepts and explore their intimate relationship.
Ionization Energy: Stripping Electrons from Atoms
Ionization energy measures the energy required to remove an electron from an atom. The higher the ionization energy, the stronger the attraction between the nucleus and the electron. This means that atoms with higher ionization energies are less likely to lose electrons.
Electron Affinity: Welcoming Electrons to the Fold
Electron affinity, on the other hand, measures the energy change when an atom accepts an electron. A positive electron affinity indicates that the atom favors gaining electrons, while a negative electron affinity indicates a reluctance to do so.
A Twist in the Dance: The Connection Between Ionization and Electron Affinity
Ionization energy and electron affinity are intertwined like two sides of the same coin. Atoms with low ionization energies tend to have high electron affinities, while atoms with high ionization energies tend to have low electron affinities.
This inverse relationship stems from the fact that removing an electron from an atom makes it more positively charged, attracting electrons more strongly. Conversely, adding an electron to an atom makes it more negatively charged, reducing its attraction for additional electrons.
Implications for Atomic Behavior
The interplay between ionization energy and electron affinity has profound implications for atomic behavior. Atoms with low ionization energies and high electron affinities are likely to form ionic bonds, where electrons are transferred from one atom to another. In contrast, atoms with high ionization energies and low electron affinities favor covalent bonds, where electrons are shared between atoms.
Unveiling the Periodic Trends
As you move across the periodic table, ionization energy generally increases from left to right and decreases from top to bottom. Electron affinity follows an opposite trend, increasing from left to right and decreasing from top to bottom.
These trends reflect the changes in the atomic radius and nuclear charge. Larger atoms have lower ionization energies due to the increased distance between the nucleus and the electrons. Conversely, atoms with high nuclear charges have higher ionization energies because of the stronger electrostatic attraction between the nucleus and the electrons.
Electronegativity: A Measure of Electron Attraction
Electronegativity is a fundamental property of atoms that describes their ability to attract electrons towards them. It plays a crucial role in determining the behavior of atoms in chemical reactions and shaping the properties of molecules and compounds.
Understanding Electronegativity
Electronegativity is a measure of how strongly an atom pulls electrons in a chemical bond. It is influenced by two key factors:
- Nuclear Charge: The greater the number of protons in an atom’s nucleus, the stronger its attraction for electrons.
- Atomic Radius: The smaller the atomic radius, the closer electrons are to the nucleus and the stronger the electrostatic attraction.
Relationship to Electron Affinity and Atomic Radius
Electronegativity is closely related to electron affinity and atomic radius. Electron affinity refers to the energy change when an atom gains an electron. The higher the electron affinity, the more the atom attracts electrons. Generally, elements with high electronegativities have high electron affinities and small atomic radii.
Electronegativity and Chemical Bonding
Electronegativity influences the type of chemical bonds formed between atoms. When two atoms with similar electronegativities bond, they form a covalent bond, where electrons are shared equally. When atoms with dissimilar electronegativities bond, they form an ionic bond, where one atom transfers electrons to the other, resulting in the formation of ions.
Applications of Electronegativity
Electronegativity is a versatile property that finds applications in various fields:
- Predicting Chemical Reactivity: It helps in understanding the reactivity of elements and the formation of chemical compounds.
- Designing Materials: Electronegativity guides the design of materials with specific properties, such as semiconductors and catalysts.
- Understanding Biological Processes: It plays a role in determining the behavior of biomolecules, such as proteins and DNA.
Electron Affinity: The Energy Dance of Electron Attraction
Picture this: an atom, like a tiny sphere, surrounded by a buzzing cloud of electrons. These electrons are like mischievous dancers, pirouetting around the atom’s nucleus like a celestial ballet.
Enter electron affinity, the energy change that occurs when an atom gains an electron. It measures the atom’s eagerness to welcome this new addition to its electron cloud. The higher the electron affinity, the more the atom desires this extra electron.
Electron affinity is like the inverse of ionization energy, which measures the energy required to remove an electron from an atom. Just as ionization energy tells us how tightly the atom holds onto its electrons, electron affinity reveals the atom’s appetite for capturing electrons.
Interestingly, electron affinity and ionization energy are closely interconnected. An atom with a high ionization energy typically has a low electron affinity, indicating its reluctance to both lose and gain electrons. On the other hand, an atom with a low ionization energy usually exhibits a high electron affinity, suggesting its eagerness to both gain and lose electrons.
Electron affinity plays a crucial role in shaping the chemical properties of elements. It governs the formation of negative ions, determines the stability of ionic compounds, and influences the reactivity of elements in chemical reactions. By understanding electron affinity, we gain insights into the intricate dance of electrons that underlies the world around us.
Valence Electrons and the Octet Rule: Uncovering the Secrets of Chemical Bonding
In the realm of chemistry, valence electrons play a pivotal role in shaping the behavior and properties of elements. These electrons occupy the outermost energy level of an atom and determine its chemical reactivity.
The octet rule, a fundamental principle in chemistry, states that atoms tend to gain, lose, or share electrons until they have eight valence electrons, forming a stable electron configuration. This rule is driven by the desire of atoms to achieve an inert gas configuration, which has a full valence shell of eight electrons.
For instance, sodium (Na) has one valence electron and tends to lose it to form positively charged sodium ions (Na+). Chlorine (Cl), on the other hand, has seven valence electrons and readily gains one electron to form negatively charged chloride ions (Cl-). By sharing valence electrons, atoms can form covalent bonds, where pairs of electrons are shared between atoms. This process creates molecules, stable units of matter with unique properties.
The octet rule is not always followed, especially in elements from the third and later periods of the periodic table. These elements can exhibit expanded valence shells, accommodating more than eight valence electrons. Phosphorus (P), for example, can form stable molecules with five valence electrons, while sulfur (S) can form stable molecules with six valence electrons.
Understanding valence electrons and the octet rule is essential for comprehending chemical bonding and predicting the behavior of elements. By knowing the number of valence electrons an atom has, chemists can infer its reactivity and the types of bonds it can form.
Metallic Character: The Tendency to Lose Electrons
In the realm of chemistry, elements dance with an intriguing ability to lose or gain electrons, giving rise to their distinct character and behavior. Among them, metals shine as the masters of electron surrender, embodying the essence of metallic character.
Metallic character, a fundamental property of elements, reflects their readiness to shed their outer electrons, rendering them positively charged ions. This ability is closely intertwined with another captivating attribute: electropositivity. The more electropositive an element, the greater its willingness to part ways with its electrons.
Interestingly, metallic character also holds a deep connection with reducing power, a measure of an element’s tendency to donate electrons in chemical reactions. As reducing power increases, so does the ability to lose electrons, further enriching the metallic character of an element.
In essence, metallic character represents the inherent drive of certain elements to reduce other substances by transferring their electrons. This remarkable property forms the foundation of numerous chemical processes and technological applications, making it an indispensable concept in the world of chemistry. Understanding metallic character unlocks a deeper appreciation for the intricate interplay of elements and their pivotal role in shaping the chemical tapestry of our world.
Non-Metallic Character: The Tendency to Gain Electrons
Among the myriad elements that make up the tapestry of our universe, non-metals stand out due to their proclivity for acquiring electrons. This enigmatic characteristic is deeply intertwined with their electronegativity and oxidizing power.
Understanding Electronegativity
Electronegativity, a measure of an atom’s ability to attract electrons, plays a crucial role in shaping non-metallic character. Non-metals possess high electronegativity values, indicating their strong urge to draw electrons towards themselves. This electron-attracting tendency makes them eager to form bonds with other atoms, especially metals.
Electron Affinity and Oxidizing Power
Electron affinity, the energy released when an atom gains an electron, complements electronegativity in shaping non-metallic character. Non-metals generally exhibit high electron affinities, meaning they readily accept electrons into their outermost energy level. This electron-accepting ability translates into their strong oxidizing power.
As oxidizing agents, non-metals tend to accept electrons from other atoms or molecules, causing them to lose electrons and become oxidized. This electron-withdrawing capability makes non-metals corrosive and reactive, contributing to their ability to participate in a wide range of chemical reactions.
Consequences of Non-Metallic Character
The non-metallic character of an element influences its various properties. These elements are often found in gaseous or solid form at room temperature. They exhibit dullness and low electrical conductivity due to their poor ability to conduct electricity. Additionally, their weak metallic bonds make them brittle and non-malleable.
In summary, non-metallic character is a defining trait of elements that avidly attract electrons. Their high electronegativity, electron affinity, and oxidizing power contribute to their unique properties, making them essential components of countless chemical processes and applications.