Electron Transport Chain (Etc): The Essential Pathway For Energy Production
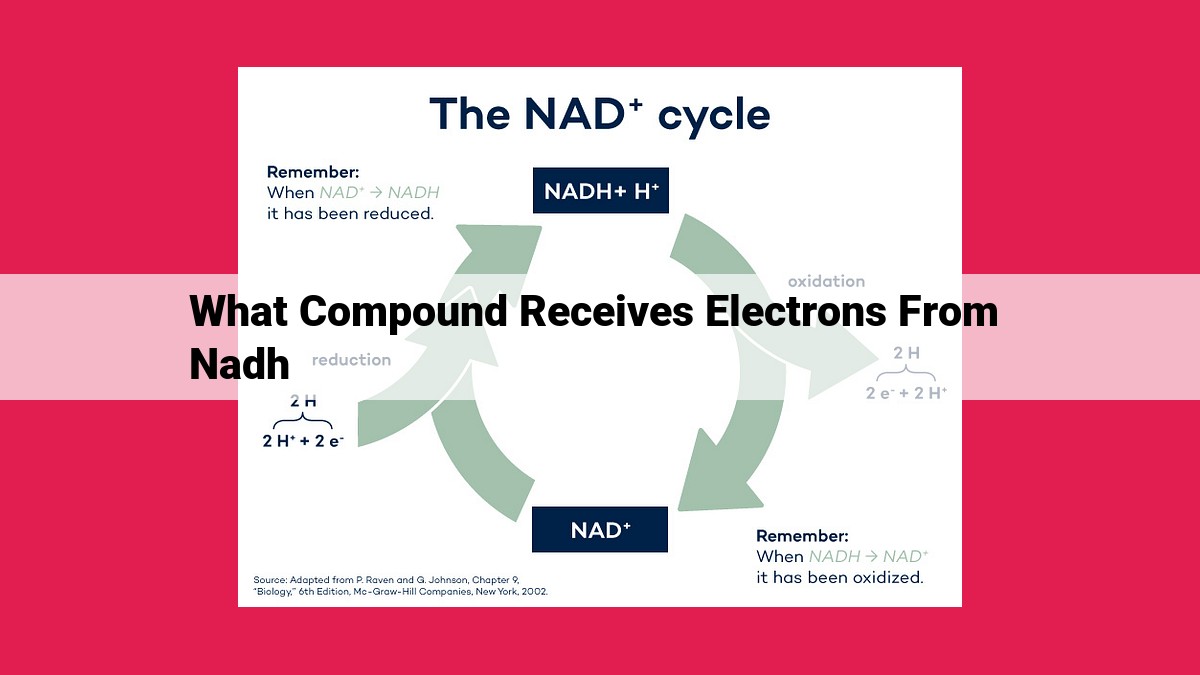
In the Electron Transport Chain (ETC), NADH molecules donate electrons to Complex I, initiating the electron transfer process. The electrons then pass through a series of protein complexes and electron carriers (such as cytochrome c, ubiquinone, and oxygen) to reach the final electron acceptor, oxygen.
The Electron Transport Chain: The Powerhouse of Cellular Respiration
Imagine yourself as a tiny molecule, eager to deliver energy to the cells in your body. As you enter the bustling metropolis of the mitochondrion, you encounter the electron transport chain (ETC), a maze-like assembly line that will transform you into life-sustaining ATP.
Nestled within the inner mitochondrial membrane, the ETC is a cellular powerhouse, generating most of the energy your cells crave. It’s made up of a series of protein complexes, each like a relay runner, passing electrons from one to another.
As you start your journey at Complex I, you’re accompanied by cytochrome c, a protein carrier that will faithfully escort you from Complex III to Complex IV. Along the way, ubiquinone steps up, transporting electrons from Complexes I and II to Complex III, ensuring the smooth flow of energy.
Your final destination is Complex IV, where oxygen eagerly awaits to accept electrons and complete the chain reaction. As electrons leap from carrier to carrier, they release energy, which is channeled into the creation of ATP.
The proton gradient builds up as electrons cascade down the ETC, creating a reservoir of energy. This pressure drives the rotation of ATP synthase, a molecular turbine that harvests the energy to generate ATP, the cellular currency of energy.
In conclusion, the ETC is the heart of cellular respiration, a masterful machine that converts the energy stored in nutrients into the ATP that fuels every facet of our existence.
Components of the Electron Transport Chain: A Journey Through the Energy-Generating Machine
At the heart of cellular respiration lies a remarkable molecular assembly known as the Electron Transport Chain (ETC). This intricate network of protein complexes orchestrates the final steps of cellular energy production, yielding the vital currency of life: ATP.
The ETC resides within the inner membrane of mitochondria, the powerhouses of our cells. It comprises four protein complexes (Complexes I-IV) that act as a relay team, passing electrons along a downhill path, like a cascading waterfall. Each complex pumps protons across the membrane, creating an electrochemical gradient that drives ATP synthesis.
Complex I: The Gatekeeper
The initial electron donor to the ETC is NADH, a molecule generated during the breakdown of glucose. Complex I, also known as NADH-coenzyme Q reductase, accepts electrons from NADH and passes them onto ubiquinone, a small, lipid-soluble electron carrier.
Complex II: An Alternative Entry Point
FADH2, another electron carrier produced during the breakdown of glucose, can also feed electrons into the ETC. Complex II, also known as succinate dehydrogenase, accepts electrons from FADH2 and transfers them to ubiquinone.
Complex III: The Electron Highway
Ubiquinone, now carrying electrons from both Complex I and II, delivers them to Complex III, or cytochrome bc1 complex. This complex oxidizes ubiquinone to ubiquinol, which then transports protons across the membrane.
Complex IV: The Final Destination
The final electron acceptor in the ETC is oxygen, the very air we breathe. Complex IV, or cytochrome c oxidase, accepts electrons from cytochrome c, a small, highly mobile protein that ferries electrons between complexes. The reduction of oxygen by Complex IV leads to the formation of water as a byproduct, releasing a surge of energy that drives proton pumping.
A Symphony of Electron Transfer
The ETC is a masterpiece of molecular engineering, a symphony of electron transfer that generates ATP, the fuel that powers cellular processes. Each protein complex plays a crucial role in this intricate dance, ensuring a steady supply of energy to sustain life’s essential functions.
**Cytochrome c: The Unsung Hero of Cellular Energy Production**
In the intricate dance of cellular respiration, there lies a protein that plays a crucial yet often unheralded role: cytochrome c. It’s a small, yet mighty molecule that shuttles electrons, the essential fuel of life, between protein complexes in the electron transport chain (ETC).
Cytochrome c is a heme protein, meaning it contains an iron ion bound to a porphyrin ring. This iron ion undergoes a redox reaction, shifting between oxidized and reduced states as it accepts and donates electrons.
**Structure and Function of Cytochrome c**
Cytochrome c is a water-soluble protein that consists of a single polypeptide chain folded into a compact globular structure. It has a negatively charged surface and a hydrophobic interior.
The heme group is located in a crevice on the surface of the protein, where it is protected from the aqueous environment. The iron ion in the heme group can bind to electrons or oxygen molecules, depending on its oxidation state.
**Role in Electron Transfer**
Cytochrome c plays a vital role in the third complex of the ETC, Cytochrome bc1 Complex. It accepts electrons from cytochrome c1 and transfers them to cytochrome oxidase in the fourth and final complex.
As it passes through the membrane, cytochrome c undergoes a conformational change that allows it to bind to cytochrome oxidase. This interaction triggers the reduction of oxygen to form water, releasing a substantial amount of energy.
Cytochrome c, though a small and often overlooked molecule, is an integral part of cellular respiration. Its ability to transfer electrons between protein complexes in the ETC is essential for the generation of adenosine triphosphate (ATP), the primary energy currency of cells. Without cytochrome c, the ETC would grind to a halt, and cells would be unable to function.
So, next time you think about cellular respiration, don’t forget the humble cytochrome c. It’s a small protein with a big job, and without it, life as we know it would not be possible.
The Electron Transport Chain: Oxygen’s Vital Role
In the bustling city of our cells, there exists a remarkable energy powerhouse known as the electron transport chain (ETC). Like a well-coordinated orchestra, the ETC orchestrates a series of electron transfers, culminating in the production of ATP, the fuel that powers our cellular activities.
Oxygen’s Grand Finale
At the end of the ETC’s electron-shuffling symphony, oxygen takes center stage as the final electron acceptor. It eagerly receives these electrons, marking the culmination of their energy-laden journey. As oxygen embraces the electrons, it undergoes a chemical transformation, combining with protons (H+) to form water.
This seemingly simple reaction is, in fact, an energy-releasing event. The energy released during the formation of water is cleverly harnessed to pump protons across the mitochondrial membrane, creating an electrochemical gradient. This gradient, like a miniature waterfall, provides the driving force for the synthesis of ATP.
ATP: The Ultimate Prize
As protons tumble down their electrochemical gradient, they encounter _ATP synthase, a molecular turbine that spins like a top. This spinning motion harnesses the energy of the proton flow to transform ADP (adenosine diphosphate) into ATP (adenosine triphosphate). ATP, the universal energy currency of cells, is then ready to power a myriad of cellular processes, fueling everything from muscle contractions to nerve impulses.
The Significance of Oxygen
The role of oxygen as the final electron acceptor in the ETC cannot be overstated. Without oxygen, the electron transport chain would grind to a halt, and the production of ATP would cease. Our cells would be left energy-starved, unable to perform their essential functions.
Thus, oxygen, the breath of life, plays an indispensable role in cellular respiration, ensuring a steady supply of ATP to keep the cellular city humming with energy.
Ubiquinone: The Electron Highway in Cellular Respiration
Meet ubiquinone, a crucial molecule in the Electron Transport Chain (ETC), the energy powerhouse of our cells. This enigmatic molecule serves as an electron shuttle, carrying electrons from Complexes I and II to Complex III like a bustling courier on a busy highway.
Structure and Function of Ubiquinone
Ubiquinone is a small, lipid-soluble molecule resembling a vitamin. Its unique structure features a long, flexible side chain attached to a quinone ring with alternating single and double bonds. This structure allows the molecule to move freely within the mitochondrial membrane and efficiently transport electrons.
Role in Electron Transfer
Ubiquinone plays a pivotal role in the ETC by transferring electrons from Complex I and II to Complex III. Complex I accepts electrons from NADH, while Complex II accepts electrons from FADH2. Ubiquinone, acting as the middleman, receives these electrons and carries them to Complex III, continuing the electron flow in the chain.
Ubiquinone is an indispensable component of the ETC, ensuring the smooth transfer of electrons from Complex I and II to Complex III. Its ability to move freely within the mitochondrial membrane and effectively transport electrons makes it a crucial cog in the energy-generating machinery of our cells.
ATP Synthesis via Proton Gradient: Acellular Energy’s Hidden Powerhouse
The Electron Transport Chain (ETC), a bustling hub within our mitochondrial powerhouses, orchestrates the final steps of cellular respiration, the process that fuels our every move. One crucial role the ETC plays is the generation of ATP, the energy currency of our cells. This ATP synthesis hinges on a meticulously crafted proton gradient, a clever trick that has revolutionized cellular energy production.
The Proton Pump: A Molecular Magic Trick
Imagine the ETC as a group of molecular pumps, each diligently working to shift protons, tiny positively charged ions, across the inner mitochondrial membrane. As electrons dance along the ETC’s protein complexes, they release energy, which is harnessed to drive these pumps.
The Proton Gradient: A Reservoir of Potential Energy
With each pumped proton, a divide forms, creating a proton gradient across the membrane. This gradient is no mere quirk; it is a carefully cultivated reservoir of potential energy, a molecular dam just waiting to unleash its power.
ATP Synthase: The Turbine of Cellular Energy
Now, enter ATP synthase, a molecular turbine strategically positioned within the mitochondrial membrane. Its job is to harness the potential energy stored in the proton gradient and convert it into the cellular gold we crave: ATP.
As protons rush back down the gradient, driven by their magnetic pull towards the mitochondrial matrix, they funnel through ATP synthase’s turbine-like structure. This movement triggers a series of conformational changes within the enzyme, ultimately leading to the synthesis of ATP from ADP and inorganic phosphate.
A Masterstroke of Energy Efficiency
The ATP synthesis via proton gradient is a testament to nature’s masterful engineering. This intricate process allows our cells to efficiently convert the energy released from electron transfer into a usable form, ATP. It is the final step in the cellular respiration saga, the grand finale that powers our every action and fuels the vibrant symphony of life.