Understanding Electron Orbitals: A Guide To Quantum Numbers And Electron Distribution
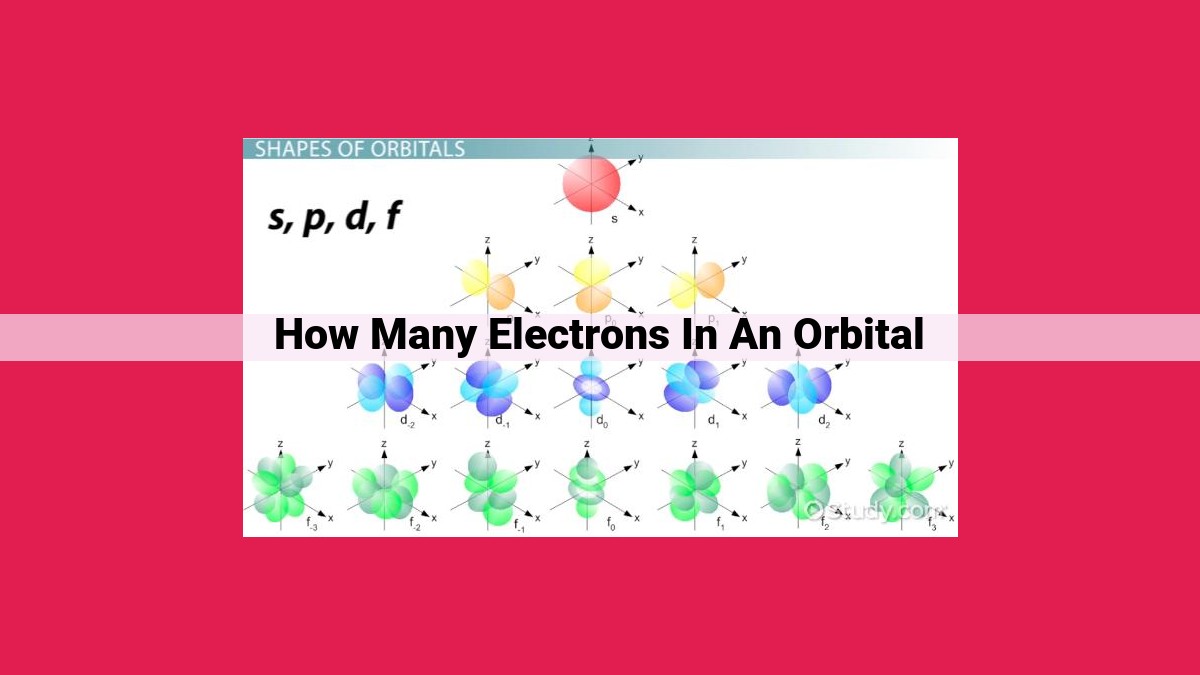
Orbitals, defined by quantum numbers, represent the regions around the nucleus where electrons are likely to be found. The number of electrons in an orbital is determined by Pauli’s Exclusion Principle and Hund’s Rule. Each orbital can hold a maximum of two electrons with opposite spins. The number of electrons in an orbital is given by 2n², where n is the principal quantum number, which represents the energy level and size of the orbital. For example, s orbitals (n=1) can hold a maximum of 2 electrons, p orbitals (n=2) can hold up to 6 electrons, d orbitals (n=3) can hold up to 10 electrons, and f orbitals (n=4) can hold up to 14 electrons.
Understanding the Basics of Orbitals and Electron Configuration
- Provide a clear introduction to the concept of orbitals and electron configuration.
- Explain the significance of quantum numbers in defining the energy and shape of orbitals.
Understanding the Building Blocks of Matter: Orbitals and Electron Configuration
In the realm of chemistry and physics, the fundamental understanding of the structure of atoms is essential. At the heart of this structure lie orbitals and electron configuration, concepts that define the energy levels and arrangement of electrons within an atom.
The Quantum World of Orbitals and Electron Configuration
Imagine an atom as a miniature solar system. Just as planets orbit a star, electrons orbit the nucleus, the dense core of an atom. However, unlike planets, electrons do not follow circular paths but reside in orbitals, regions of space with a high probability of finding them.
The properties of an orbital are determined by a set of quantum numbers. These numbers describe an electron’s energy (n), shape (l), orientation in space (ml), and spin (ms). The first three quantum numbers determine the orbital’s shape and energy level.
The Pauli Exclusion Principle and Spin
The Pauli Exclusion Principle dictates that no two electrons can occupy the same quantum state simultaneously. This principle ensures that each orbital can accommodate a maximum of two electrons, with their spins aligned in opposite directions. Spin, an intrinsic angular momentum, can be either spin up or spin down.
Hund’s Rule and Magnetism
Hund’s Rule predicts how electrons will fill orbitals. It states that electrons will occupy degenerate orbitals (orbitals with the same energy) with parallel spins before pairing up. This spin alignment can result in unpaired electrons, which contribute to the atom’s magnetic properties.
Unveiling the Secrets of Pauli’s Exclusion Principle and Electron Spin
In the realm of quantum mechanics, electrons dance in a world of whirling energy, each occupying a unique quantum state. This enigmatic choreography is governed by two fundamental principles: Pauli’s Exclusion Principle and the concept of electron spin.
Pauli’s Exclusion Principle: No Two Electrons are Twins
Imagine a universe where no two electrons are identical. This is the essence of Pauli’s Exclusion Principle. According to this principle, no two electrons can exist in the same quantum state within the same atom. Each quantum state is defined by a unique set of four quantum numbers, including energy level, shape, orientation, and spin.
This principle ensures the individuality of electrons, preventing them from merging into indistinguishable particles. It’s like the cosmic law that governs electron behavior, prohibiting them from sharing the same “space” in the atomic realm.
Electron Spin: The Electron’s Inner Dance
Electrons are not merely static entities. They possess an intrinsic angular momentum called spin, which can be visualized as a tiny spinning top. Spin endows electrons with two possible orientations, which are labeled as “spin up” and “spin down.”
The spin of an electron is a fundamental property that cannot be changed. It’s as if electrons carry an invisible compass, guiding their behavior and interactions with other electrons.
The Harmony of Pauli and Spin: Unraveling Magnetism
Pauli’s Exclusion Principle and electron spin work in concert to determine the magnetic properties of atoms. When unpaired electrons with the same spin occupy an atomic orbital, they create a magnetic field. This is the basis of magnetism in materials.
For instance, iron atoms have unpaired electrons, making them magnetic. The alignment of these unpaired spins in the same direction creates domains that give iron its magnetic properties.
The Pauli Exclusion Principle and electron spin are fundamental concepts that govern the behavior of electrons, shaping the very fabric of our universe. These principles not only ensure the uniqueness of electrons but also influence the magnetic properties of materials. Understanding these concepts is essential for unraveling the mysteries of quantum mechanics and the behavior of matter at its most fundamental level.
Exploring Hund’s Rule and Its Influence on Magnetism
Understanding Hund’s Rule
Hund’s Rule is a fundamental principle in quantum mechanics that governs the arrangement of electrons within an atomic orbital. It states that the lowest energy configuration for a set of electrons in degenerate orbitals is the one in which the electrons have the maximum possible number of unpaired spins. In other words, electrons prefer to occupy different spin states before pairing up.
Mechanism of Hund’s Rule
This behavior can be attributed to an effect known as exchange repulsion. When electrons have parallel spins, they experience a repulsive force due to their identical magnetic fields. This repulsion is minimized when electrons have antiparallel spins, allowing them to occupy the same orbital while maintaining a lower energy state.
Magnetic Fields and Unpaired Electrons
The presence of unpaired electrons has a profound impact on the magnetic properties of atoms. Electrons with unpaired spins generate tiny magnetic fields due to their intrinsic angular momentum. When multiple unpaired electrons are present in an atom, their magnetic fields align, creating a net magnetic moment. This magnetic moment makes atoms susceptible to external magnetic fields, leading to phenomena such as paramagnetism and ferromagnetism.
Importance for Chemical Properties
Hund’s Rule plays a crucial role in determining the valence electrons of an atom, which are the electrons involved in chemical bonding. The number of unpaired electrons in the outermost energy level influences the chemical reactivity and bonding behavior of the element. Elements with unpaired electrons tend to be more reactive, forming stable compounds with other atoms.
Examples of Hund’s Rule
The following examples illustrate Hund’s Rule:
- Oxygen has two unpaired electrons in its outermost p-orbital, giving it a paramagnetic character.
- Iron has four unpaired electrons in its d-orbitals, resulting in a ferromagnetic material.
- Helium has two paired electrons in its s-orbital, making it diamagnetic (not susceptible to magnetic fields).
Delving into the Types of Atomic Orbitals
- Introduce the different types of atomic orbitals: s, p, d, and f.
- Provide a detailed description of their shapes, nodal planes, and energy levels.
Unveiling the Enigmatic World of Atomic Orbitals
In the realm of quantum mechanics, electrons inhabit mysterious spaces known as orbitals. Visualize them as ethereal clouds enveloping the atomic nucleus, where the electrons reside, dictating the atom’s behavior and properties.
Types of Atomic Orbitals: The Quartet of Shapes
There are four main types of atomic orbitals, each with a distinct shape:
- s orbitals: These are spherical clouds, the simplest of the bunch. Electrons in s orbitals are close to the nucleus and possess the lowest energy.
- p orbitals: Resembling dumbbells, p orbitals exist in three orientations along the x, y, and z axes. Their energy levels are slightly higher than s orbitals.
- d orbitals: These complex orbitals come in five different shapes, characterized by lobes and nodal planes. They have higher energy levels than p orbitals.
- f orbitals: The most exotic of the orbitals, f orbitals possess intricate shapes with multiple lobes. They are found in elements with atomic numbers greater than 57.
Nodal Planes: The Boundaries of Electron Probability
Nodal planes are surfaces within orbitals where the probability of finding an electron is zero. Imagine slicing through an orbital like a cake, revealing the regions where electrons are likely (or unlikely) to reside. These planes help define the orbital’s unique shape.
Energy Levels: The Staircase of Electron Excitation
Orbitals possess different energy levels, with s orbitals having the lowest energy and f orbitals the highest. Electrons occupy orbitals with the lowest energy first, following a staircase pattern. When an electron absorbs energy, it can jump to an orbital with a higher energy level, becoming excited.
The types of atomic orbitals, with their distinct shapes, nodal planes, and energy levels, play a pivotal role in determining an atom’s properties and behavior. They are the building blocks of the microscopic world, shaping the interactions between elements and governing the chemical reactions that shape our universe.
Shedding Light on Valence Electrons: The Key Players in Chemical Bonding
In the realm of chemistry, understanding the behavior of electrons is crucial for deciphering the intricate world of chemical bonding. Valence electrons, the outermost electrons in an atom’s electronic structure, hold the key to unraveling these intricate interactions.
The Dance of Valence Electrons: Bonding’s Invisible Choreographers
Valence electrons, like graceful dancers on a molecular stage, determine an atom’s chemical reactivity and bonding capabilities. They are the gatekeepers of chemical interactions, orchestrating the formation and breaking of bonds between atoms. The arrangement of valence electrons in an atom’s outermost energy level, known as the valence electron configuration, plays a pivotal role in shaping its chemical properties.
The Periodic Table: A Map of Valence Electron Patterns
The Periodic Table, a chemist’s guide to the elements, organizes elements based on their valency, or the number of valence electrons they possess. Elements within the same group or period share similar valence electron configurations and, consequently, exhibit similar chemical behaviors.
For instance, the noble gases, nestled on the far right of the Periodic Table, boast a full complement of valence electrons, making them chemically inert. On the other hand, highly reactive alkali metals, residing on the left, possess a single valence electron, eagerly seeking to shed it in order to achieve stability.
Determining the Number of Electrons in an Orbital
As we delve deeper into the fascinating world of quantum mechanics, it’s essential to grasp how to determine the maximum number of electrons that can reside within an orbital. This knowledge forms the cornerstone of understanding the electronic structure of atoms and their subsequent behavior.
Quantum Numbers: Guiding the Electron’s Fate
Like celestial bodies orbiting a star, electrons occupy specific orbitals around the atomic nucleus, each with a unique set of properties. These properties are defined by three quantum numbers: n, l, and ml.
n represents the principal energy level, dictating the electron’s distance from the nucleus. Higher n values correspond to orbitals farther from the nucleus and higher energy levels.
l describes the orbital’s shape. It can take values from 0 to n-1. Different values of l give rise to s, p, d, and f orbitals, each with distinctive spatial orientations.
ml represents the magnetic quantum number and describes the orbital’s orientation in space. For a given l value, ml can range from -l to +l.
Maximum Occupancy: A Matter of Spin
The maximum number of electrons that can occupy an orbital is governed by the Pauli Exclusion Principle. This principle states that no two electrons can occupy the same quantum state simultaneously. Consequently, each orbital can accommodate a maximum of two electrons, provided they have opposite spins.
Spin, denoted by the quantum number ms, describes the electron’s intrinsic angular momentum. Electrons can have either spin up or spin down, represented by +1/2 and -1/2, respectively.
s Orbitals: A Lone Wolf’s Domain
s orbitals have l = 0, indicating a spherical shape. They can accommodate a maximum of two electrons, both with opposite spins.
p Orbitals: Trio of Possibilities
p orbitals have l = 1, resulting in three dumbbell-shaped lobes oriented along the x, y, and z axes. Each lobe can house two electrons, leading to a maximum capacity of six electrons per p orbital.
d Orbitals: A Quintet of Options
d orbitals have l = 2, giving rise to five intricate shapes. They can accommodate a maximum of ten electrons, with each of the five lobes holding a pair of opposite-spin electrons.
f Orbitals: The Grand Finale
f orbitals have l = 3, resulting in a complex set of seven shapes. They can accommodate a maximum of fourteen electrons, distributed among the seven lobes.
Determining the number of electrons in an orbital requires an understanding of the quantum numbers that define its properties. By considering the principal energy level, orbital shape, and magnetic quantum number, we can accurately predict the maximum electron occupancy of any orbital. This knowledge is pivotal for unraveling the complexities of atomic structure and its implications for chemistry and materials science.