Understanding Electron Configuration: Insights Into The Chemical Nature Of Aluminum
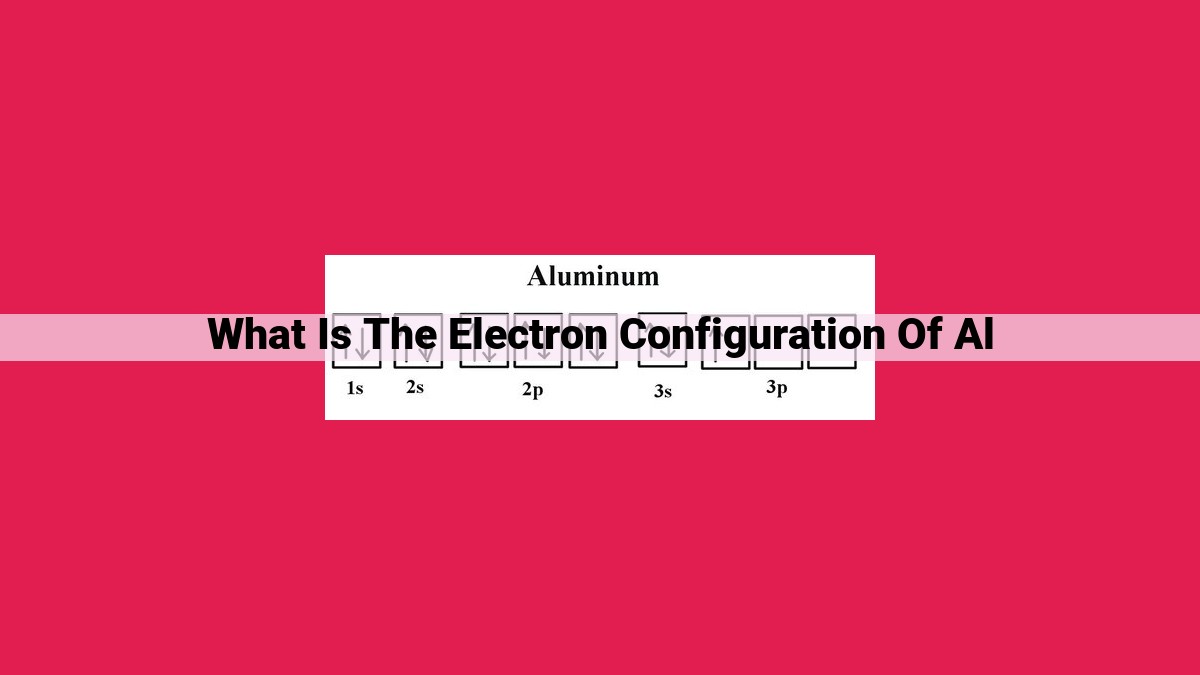
The electron configuration of an element describes the arrangement and distribution of electrons in its atomic orbitals. Aluminum (Al), with an atomic number of 13, has an electron configuration of 1s²2s²2p⁶3s²3p¹. This means that it has two electrons in the first energy level (n=1), eight electrons in the second energy level (n=2), and three electrons in the third energy level (n=3). The configuration of Al provides insights into its chemical properties, such as its high electronegativity and low ionization energy, which make it reactive and prone to form ionic bonds.
Electron Configuration: Unveiling the Secrets of Chemical Elements
Just like a fingerprint distinguishes each individual, every element on the periodic table possesses a unique identity defined by its electron configuration. This intricate blueprint reveals the arrangement of electrons within an atom, providing invaluable insights into its chemical properties and behavior.
Electrons: The Building Blocks of Matter
Atoms, the fundamental building blocks of all matter, are composed of a dense nucleus surrounded by a cloud of orbiting electrons. Understanding the arrangement of these electrons is crucial to comprehending an element’s chemical nature.
Electron Configuration: A Map to the Atomic Landscape
Electron configuration depicts the distribution of electrons across various energy levels, each represented by a specific quantum number. These numbers provide a roadmap to the electrons’ precise location and energy within the atom.
Quantum Numbers: The Guiding Principles
Four fundamental quantum numbers govern the behavior of electrons:
- Principal Quantum Number (n): Indicates the energy level or shell, with higher numbers denoting farther shells from the nucleus.
- Azimuthal Quantum Number (l): Defines the shape of the orbital, designated by letters: s, p, d, and f.
- Magnetic Quantum Number (ml): Describes the orientation of the orbital in space, with multiple orbitals sharing the same energy level.
- Spin Quantum Number (ms): Represents the intrinsic spin of the electron, either “up” or “down.”
Orbitals: The Electron’s Home Address
Based on their quantum numbers, electrons occupy specific regions within the atom known as orbitals. Each orbital has a unique shape and can accommodate a maximum number of electrons.
Electron Configuration Notation: Translating the Atom’s Identity
Electron configurations are represented using a concise notation that indicates the energy levels, orbitals, and number of electrons in each. This notation serves as the “chemical fingerprint” of each element.
Quantum Numbers: The Building Blocks of Electron Configuration
In the vast and intricate realm of chemistry, understanding the arrangement of electrons within an atom is crucial to unraveling the mysteries of its behavior. This intricate dance is governed by a set of fundamental principles known as quantum numbers, providing a blueprint for the symphony of electron distribution.
Principal Quantum Number (n): The Orbital’s Floor
The principal quantum number governs the energy level of the electron’s orbital, akin to the floors of a multi-storied building. The higher the value of n, the farther the orbital is from the nucleus, and thus the higher its energy.
Azimuthal Quantum Number (l): The Orbital’s Shape
The azimuthal quantum number determines the shape of the orbital, resembling the blueprint for an architect’s design. It defines the type of orbital, such as s, p, d, or f, each possessing a unique spatial distribution.
Magnetic Quantum Number (ml): The Orbital’s Orientation
The magnetic quantum number specifies the orientation of the orbital within its energy level, akin to the different rooms within a suite. It determines the number of orbitals with the same energy that coexist within a single energy level.
Spin Quantum Number (ms): The Electron’s Intrinsic Spin
The spin quantum number represents the intrinsic spin of the electron, akin to the rotation of a spinning top. It can take two values, +1/2 or -1/2, indicating the two possible orientations of the electron’s spin.
These quantum numbers, like the invisible architects of the atomic realm, dictate the behavior and properties of electrons within an atom. They are the fundamental building blocks upon which the intricate tapestry of electron configuration is woven.
Orbitals: The Electron’s Unique Abode
Imagine electrons as tiny dancers, each occupying a specific spot on the chemical stage. These spots are not random but follow a set of rules, much like the choreography in a ballet. Enter the world of orbitals, the designated addresses for electrons.
Types of Orbitals: A Hierarchy of Shapes
Based on the quantum numbers, electrons reside in distinct types of orbitals, categorized as s, p, d, and f. Each type has a unique shape that determines the electron’s movement.
- s orbitals: Picture a spherical cloud surrounding the nucleus, like a cozy bubble where the electron can dance freely.
- p orbitals: These are dumbbell-shaped, with two lobes pointing in opposite directions like two wings.
- d orbitals: More complex than p orbitals, d orbitals exhibit four lobes arranged in different orientations.
- f orbitals: The most elaborate of all, f orbitals have eight lobes with intricate shapes.
Electron Capacity: How Many Guests Each Orbital Can Host
Like any party space, orbitals have a maximum capacity for electrons. Each s orbital can accommodate two electrons, while p orbitals can hold up to six (two in each lobe). D orbitals have a capacity of ten electrons, and f orbitals can house fourteen.
Electron Configuration: A Matter of Order
The arrangement of electrons in orbitals follows a specific order based on their energy levels. Electrons always prefer the lowest energy state, so they fill the orbitals in a bottom-up approach. This order is crucial for understanding an element’s chemical behavior.
Unveiling the Language of Electron Configuration
Step into the realm of electron configuration, a concept that holds the key to understanding the chemical properties of elements. It’s like a cosmic dance where electrons, the tiny particles that orbit atoms, follow a set of rules that define their behavior.
To represent this intricate dance, chemists have devised a special notation. It’s a language that describes how electrons occupy specific energy levels around the nucleus. Each level, like a celestial sphere, can accommodate a certain number of electrons.
The first energy level, the closest to the nucleus, is like the royal court, holding a maximum of two electrons. The next level, a bit farther out, can house up to eight electrons. And so it goes, with each level offering more space for these energetic dancers.
But here’s the catch: electrons are quirky creatures with their own set of rules. According to the Pauli exclusion principle, no two electrons can occupy the exact same energy state. They’re like siblings in a crowded room, each demanding their own personal space.
That’s where the magic of quantum numbers comes in. Each electron possesses four unique quantum numbers that describe its position and spin. These numbers are like a secret code, guiding electrons to their designated orbitals.
Orbitals, the electrons’ abodes, come in different shapes and sizes. There are spherical s-orbitals, dumbbell-shaped p-orbitals, and more complex d- and f-orbitals. Each orbital can hold a maximum of two electrons, ensuring that the electron family remains cozy and organized.
Understanding electron configuration is like having a secret decoder ring, unlocking the mysteries of chemical behavior. It reveals why elements like helium are inert loners, while sodium is eager to share its electrons.
From predicting ionization energies to understanding molecular bonding, electron configuration is a powerful tool that empowers chemists to navigate the world of atoms and molecules. It’s the foundation upon which the periodic table is built, organizing elements according to their electron configurations and uncovering their fascinating properties.
Electron Configuration: Unlocking the Secrets of Chemical Behavior
Electron configuration, a fundamental concept in chemistry, unveils the arrangement of electrons within an atom’s orbitals. This knowledge holds the key to understanding the chemical properties and behavior of elements.
Electrons and Their Quantum Home
Electrons reside in specific regions around the atom’s nucleus, called orbitals. Quantum numbers, like cosmic addresses, describe each orbital’s unique characteristics:
- Principal Quantum Number (n): The energy level and size of the orbital.
- Azimuthal Quantum Number (l): The shape of the orbital (s, p, d, f).
- Magnetic Quantum Number (ml): The orientation of the orbital in space.
- Spin Quantum Number (ms): The two possible orientations for an electron’s spin.
Aluminum’s Electron Configuration: A Case Study
Let’s delve into the electron configuration of aluminum, an element renowned for its versatility.
- Atomic Number (Z): 13
- Electron Configuration: 1s²2s²2p⁶3s²3p¹
This configuration reveals that aluminum has:
- Three energy levels (n): 1, 2, and 3
- Total of 13 electrons: The same as its atomic number
- One unpaired electron: In the 3p orbital
Implications for Aluminum’s Chemical Behavior
Aluminum’s electron configuration has profound implications for its chemical properties:
- Low ionization energy: Removing an electron from the outermost 3p orbital requires less energy.
- High electronegativity: Aluminum readily attracts electrons, forming cations in ionic compounds.
- Metallic bonding: The unpaired electron in the 3p orbital participates in metallic bonding, contributing to aluminum’s characteristic strength and ductility.
- Amphoteric behavior: Aluminum can react with both acids and bases, owing to the presence of both electropositive and electronegative sites.
Electron Configuration: A Guiding Light in Chemistry
Electron configuration is a fundamental tool in chemistry, offering insights into:
- Chemical bonding: Predicts the types of bonds an element can form.
- Molecular structure: Determines the three-dimensional arrangement of molecules.
- Materials science: Guides the design and engineering of novel materials with specific properties.
Understanding electron configuration empowers chemists to unravel the mysteries of chemical behavior, design new materials, and unlock the potential of chemistry for the advancement of science and technology.
Significance of Electron Configuration: Unlocking the Secrets of Chemistry
Electron configuration, the arrangement of electrons within an atom’s orbitals, is the cornerstone of chemistry. It profoundly influences the element’s chemical properties, paving the way for countless discoveries and applications.
Ionization Energy: The ionization energy, the energy required to remove an electron from an atom, is directly tied to electron configuration. Elements with loosely held outermost electrons (low ionization energy) tend to form cations (positively charged ions). Conversely, elements with tightly held electrons (high ionization energy) form anions (negatively charged ions).
Electronegativity: The electronegativity of an element, its ability to attract electrons in a chemical bond, is also governed by electron configuration. Elements with high electronegativity have a strong pull on electrons, forming covalent bonds with other atoms. Elements with low electronegativity form metallic bonds with weak electrostatic interactions.
Bonding: Electron configuration dictates the valence electrons, the electrons in the outermost energy level, which participate in chemical bonding. The number and arrangement of valence electrons determine the type and strength of bonds formed. For instance, elements with a full valence shell (stable configuration) are chemically inert, while those with incomplete valence shells are highly reactive.
Implications: The significance of electron configuration extends far beyond these fundamental properties. It unravels the mysteries of chemical bonding, allowing us to predict the structure and behavior of countless compounds. Electron configuration also underpins materials science, guiding the design of advanced materials with tailored properties. Furthermore, it empowers chemists to create new drugs, devise innovative energy solutions, and explore the frontiers of nanotechnology.
Applications of Electron Configuration: Unraveling the Chemistry of Matter
Electron configuration, describing the distribution of electrons in atomic orbitals, holds immense importance in understanding the chemical behavior of elements. By deciphering the electron configurations of atoms and molecules, scientists can unravel the secrets behind their chemical interactions and predict their properties.
Electron Configuration and Chemical Bonding
The electron configuration of an atom significantly influences its bonding behavior. Elements with similar electron configurations often exhibit similar chemical properties. For instance, elements in the same group of the periodic table have identical valence electron configurations, leading to similarities in their chemistry. The arrangement of electrons in orbitals determines the type of bonds an atom can form, such as ionic, covalent, or metallic bonds.
Molecular Structure and Electron Configuration
Electron configuration plays a crucial role in determining the molecular structure of compounds. The arrangement of electrons in orbitals influences the shape and polarity of molecules. For example, molecules with lone pairs of electrons often have a bent or asymmetric shape, while molecules with symmetrical electron configurations tend to be linear or planar. The electron configuration also helps predict the reactivity of molecules and their interactions with other substances.
Electron Configuration in Materials Science
In the realm of materials science, electron configuration is a key factor in designing and developing materials with desired properties. The electronic structure of materials dictates their electrical, optical, and magnetic properties. By manipulating the electron configuration of materials, scientists can engineer materials with tailored properties for specific applications, such as semiconductors, superconductors, and optoelectronic devices.
Electron configuration is a fundamental concept that unlocks the secrets of chemical behavior. Its applications span various fields of chemistry and materials science, providing valuable insights into the structure, properties, and reactivity of molecules and materials. By understanding electron configuration, scientists can harness the power of chemistry to create innovative materials and technologies that shape our world.