The Electrochemical Gradient: Understanding Ion Movement And Energy Generation In Cells
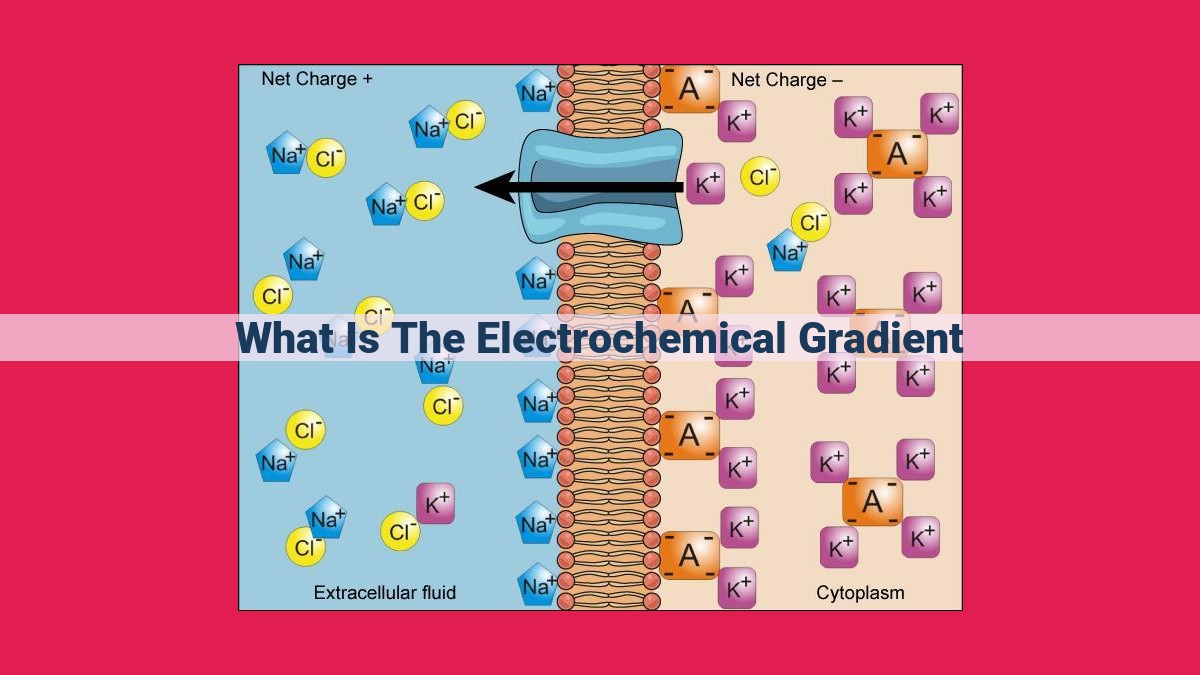
The electrochemical gradient, a driving force for ion movement across cell membranes, comprises two components: an ion concentration gradient and a membrane potential. Electrogenic pumps establish the membrane potential, while ion channels and transporters facilitate ion movement down the concentration gradient or against it using ATP. Active ion transporters use ATP to drive ions against their gradient, whereas passive transporters facilitate ion movement down their gradient. The proton-motive force generates a proton gradient used to drive ATP synthesis or other processes. Specific pumps like the sodium-potassium pump or calcium pump regulate ion concentrations and maintain membrane potential. Understanding the electrochemical gradient is crucial for cell function, as it allows selective ion movement, maintains membrane potential, and drives energy-requiring processes.
Electrochemical Gradients: The Driving Force of Ion Movement
In the symphony of life, the movement of ions plays a fundamental role, like the notes in a captivating melody. Defining the electrochemical gradient is like understanding the conductor’s baton, orchestrating the ions’ dance across biological membranes. This force, a fusion of ion concentration differences and membrane potential, drives the flow of ions, influencing everything from nerve impulses to cellular homeostasis.
Ion Concentration Differences: The Concentration Divide
Imagine a river with a steep bank on one side and a gentle incline on the other. The water flows effortlessly from the higher elevation to the lower, driven by the concentration gradient. Similarly, ions, like tiny charged particles, move from areas of higher concentration to areas of lower concentration across membranes. This concentration difference acts like a magnet, pulling ions towards the region where they are less abundant.
Membrane Potential: Electrical Allure
Adding another layer to the electrochemical gradient is the membrane potential. It’s like an invisible fence, with one side of the membrane positively charged and the other negatively charged. This electrical potential difference attracts ions of opposite charge, creating a driving force that propels them across the membrane.
The Symphony of Ion Movement
The electrochemical gradient orchestrates a harmonious interplay of ion channels, transporters, and pumps, each playing a specific role in regulating ion movement. Ion channels function like doorways, allowing ions to pass through selectively, while transporters act as carriers, shuttling ions across membranes against or with the concentration gradient. Electrogenic pumps, like tiny power plants, create the membrane potential by pumping positive ions across the membrane.
ATP: The Energy Source
Energy is the lifeblood of cellular processes, and ion movement is no exception. ATP hydrolysis, the breakdown of ATP, provides the energy needed for active transport, enabling ions to defy concentration gradients.
Unveiling the Secrets of Ion Movement
The electrochemical gradient is a complex force that underpins a vast array of biological processes. By understanding its components and mechanisms, we unlock the secrets of cell function and gain a deeper appreciation for the intricate symphony of life at the molecular level.
Components of the Electrochemical Gradient
- Ion Concentration Gradient: Explain the concentration difference of ions across a membrane and its contribution to ion movement.
- Membrane Potential: Discuss the electrical potential difference across a membrane and how it influences ion distribution.
Components of the Electrochemical Gradient: Driving Ion Movement
The electrochemical gradient, a force driving the movement of ions across cell membranes, has two key components:
Ion Concentration Gradient
Imagine a membrane separating two compartments, each containing a different concentration of ions. Like hikers descending a mountain, ions tend to move down their concentration gradient, from an area of high concentration to low concentration. This gradient creates a *concentration gradient**, a chemical force that drives ion movement.
Membrane Potential
In addition to the concentration gradient, a membrane potential exists across the membrane due to the unequal distribution of ions. Like magnets with opposite charges attracting each other, oppositely charged ions on either side of the membrane create an electrical potential. This difference in electrical potential exerts an influence on ion distribution, attracting ions to the oppositely charged side of the membrane.
Together, the ion concentration gradient and membrane potential combine to create the electrochemical gradient, a powerful force behind the selective movement of ions across cell membranes. This gradient plays a crucial role in various cellular processes, from nerve impulse transmission to maintaining cellular homeostasis and regulating cell volume.
Unlocking the Secrets of Ion Movement: Electrogenic Pumps
In the intricate world of cell biology, ion movement plays a pivotal role in various cellular processes. Understanding how ions move across cell membranes is crucial to unraveling the mechanisms behind these processes. Among the key players in this ion-orchestrated symphony are electrogenic pumps, the unsung heroes responsible for creating the electrical potential that drives ion movement.
Electrogenic pumps are specialized membrane proteins that possess the remarkable ability to transport positive ions, such as sodium (Na+) or potassium (K+), across cell membranes. As these positively charged ions move across the membrane, they create an electrical potential difference, known as the membrane potential. This potential difference, like a miniature battery, serves as the driving force for the movement of other ions across the membrane.
How Do Electrogenic Pumps Work?
Electrogenic pumps utilize a clever strategy to establish the membrane potential. They bind to specific ions on one side of the membrane, often the inside of the cell, and then transport them to the other side, the outside of the cell. During this transport process, the pumps channel the positive ions in a specific direction, essentially creating an ionic current.
This ionic current, like a tiny electrical current, sets up the membrane potential. The side of the membrane where the positive ions accumulate becomes more positive, while the opposite side becomes more negative. This electrical gradient provides the necessary driving force for other ions to move across the membrane, ensuring proper ion distribution and cellular function.
In essence, electrogenic pumps serve as the “electricians” of the cell, establishing the electrical circuit that powers ion movement. Their ability to create and manipulate the membrane potential enables cells to precisely control ion concentrations and maintain proper cell function, demonstrating the remarkable complexity and sophistication of cellular processes.
Ion Channels: Gatekeepers of Ion Movement
In the intricate realm of cellular processes, ion channels play a pivotal role in the movement of ions across cell membranes. These specialized proteins are embedded within the membrane, forming pores that allow specific ions to pass through. Understanding their function is crucial for unraveling the secrets of cellular communication and the regulation of physiological processes.
Ion channels fall into two main categories: gated and ungated. Gated channels open and close in response to specific stimuli, such as voltage changes, ligand binding, or mechanical forces. Ungated channels remain constantly open, allowing ions to flow down their concentration gradient.
Voltage-gated ion channels are particularly important in excitable cells, such as neurons and muscle cells. When the membrane potential changes, voltage-gated channels open or close, controlling the influx and efflux of ions. This change in ion movement triggers electrical signals that propagate along these cells, enabling communication and action.
Ligand-gated ion channels open in response to the binding of a specific chemical messenger, or ligand. These channels are found in many cell types and play a role in signaling pathways and sensory perception.
Mechanically-gated ion channels open when the membrane is subjected to physical forces, such as stretching or pressure. These channels are involved in sensory transduction, allowing cells to detect changes in their mechanical environment.
The diversity of ion channels ensures precise control over the movement of ions across cell membranes. By selectively allowing or blocking the passage of specific ions, these channels regulate electrical excitability, maintain ion balance, and enable a wide range of cellular processes.
Ion Transporters: The Gatekeepers of Cellular Ion Balance
Imagine a bustling city with a myriad of citizens moving in and out constantly. To maintain order and ensure smooth traffic flow, the city must regulate the movement of these individuals through strategically placed gates. Similarly, cells utilize specialized proteins called ion transporters to control the passage of ions across their membranes.
Ion transporters are classified into two main types:
Active Ion Transporters: Powering Ions Against the Odds
Active transporters are the unsung heroes of the cellular world. They defy the laws of nature by pumping ions against their concentration gradient, utilizing the energy stored in ATP. These pumps work tirelessly to establish and maintain electrochemical gradients, driving ion movement across membranes.
Examples of active transporters include the sodium-potassium pump and the calcium pump. The sodium-potassium pump maintains the proper balance of sodium and potassium ions across the cell membrane, which is crucial for regulating cell volume, nerve impulse transmission, and muscle contraction. The calcium pump, on the other hand, pumps calcium ions out of the cell to prevent excessive accumulation, which could lead to cell death.
Passive Ion Transporters: Facilitating Downhill Ion Flow
Unlike active transporters, passive ion transporters operate without directly consuming ATP. Instead, they facilitate the movement of ions down their concentration gradient, from an area of high concentration to an area of low concentration. This downhill flow occurs through channels or carriers embedded within the cell membrane.
Ion channels are selective pores that allow specific ions to pass through based on their charge and size. These channels can be either gated (controlled by stimuli such as voltage or ligand binding) or ungated (always open). Ion carriers, on the other hand, bind to specific ions and transport them across the membrane without creating pores.
Examples of passive ion transporters include potassium channels and chloride channels. Potassium channels facilitate the outflow of potassium ions from cells, contributing to the resting membrane potential. Chloride channels, on the other hand, allow chloride ions to move down their concentration gradient, playing a role in cell volume regulation and nerve impulse transmission.
The Proton-Motive Force: Unraveling the Energy Cycle of Life
In the bustling metropolis of our cells, a hidden force orchestrates the vital flow of ions, driving protons across membranes like tiny electric cars. This enigmatic force, known as the proton-motive force, plays a pivotal role in fueling some of the most fundamental processes that sustain life.
The proton-motive force arises from the electrochemical gradient across a membrane, a delicate balance between ion concentration gradients and membrane potential. As protons accumulate on one side of the membrane, creating an ion concentration gradient, an electrical potential difference also forms, pulling protons towards the opposite side. This combined force, like a tiny battery, drives the movement of protons across the membrane.
Think of the proton-motive force as a molecular engine, powering the movement of protons against their concentration gradient. This energy-intensive process is essential for maintaining the delicate pH balance within cells and organelles. Moreover, it drives the synthesis of ATP, the universal energy currency of the cell, through a process called oxidative phosphorylation.
The proton-motive force is harnessed by electrogenic pumps, such as the respiratory chain in mitochondria, which actively transport protons across membranes, creating the necessary electrochemical gradient. This gradient, in turn, fuels the movement of ions through ion channels and ion transporters, further contributing to the energy dance of the cell.
In photosynthesis, the proton-motive force drives the movement of protons across the thylakoid membrane of chloroplasts, generating the energy needed to convert carbon dioxide and water into glucose. This fundamental process sustains the very foundation of life on our planet.
Specific ion pumps, such as the sodium-potassium pump, maintain membrane potential and regulate ion concentrations within cells. These molecular gatekeepers ensure the proper function of nerve impulses, muscle contractions, and the delicate balance of fluids within our bodies.
Understanding the proton-motive force is like unlocking a secret code that governs the very essence of life. It is a testament to the intricate and dynamic nature of our cells, where energy flows through a symphony of molecular interactions, driving the countless processes that keep us alive and thriving.
Specific Ion Pumps: Guardians of Membrane Balance
The electrochemical gradient, a fundamental force in cellular life, relies on ion pumps to maintain its balance. Among these pumps, two stand out: the sodium-potassium pump and the calcium pump.
Sodium-Potassium Pump: The Membrane’s Gatekeeper
Imagine a gatekeeper at a door, controlling the flow of people between two rooms. The sodium-potassium pump, also known as the Na+/K+-ATPase pump, serves this role for ions across the cell membrane.
It actively transports three sodium ions out of the cell while bringing two potassium ions in. This process, fueled by the hydrolysis of ATP, generates an electrochemical gradient across the membrane. The resulting imbalance of sodium and potassium ions creates a membrane potential that influences the movement of other ions.
Calcium Pump: Regulating Calcium Harmony
Calcium ions are vital for many cellular processes, but their levels must be carefully controlled. The calcium pump, or Ca2+-ATPase pump, acts as a gatekeeper for calcium, keeping its levels low inside the cell.
Using ATP as its energy source, the calcium pump actively transports calcium ions out of the cell or into specific intracellular compartments. This maintains the low intracellular calcium concentration, preventing harmful calcium overload and ensuring optimal cellular function.
The sodium-potassium pump and the calcium pump are crucial ion pumps that maintain membrane balance and cellular homeostasis. They actively transport ions against their concentration gradients, using the energy from ATP to create and regulate the electrochemical gradient that drives ion movement. Understanding these pumps is essential for comprehending the fundamental principles of cellular physiology.
ATP Hydrolysis: The Energetic Fuel for Active Ion Transport
Within the intricate machinery of our cells lies a remarkable process called ATP hydrolysis, a fundamental cellular process that drives the vital movement of ions across membranes. This intricate dance of ion movement plays a pivotal role in maintaining cellular homeostasis, regulating nerve impulse transmission, and facilitating nutrient uptake.
ATP, the Cellular Energy Currency
ATP, the ubiquitous energy currency of cells, is a molecule that stores readily accessible energy. When ATP undergoes hydrolysis, the breakdown of its terminal phosphate bond releases significant energy. This stored energy fuels a variety of cellular processes, including the active transport of ions across membranes.
Active Transport: Moving Ions Uphill
Active transport, unlike passive transport, propels ions against their concentration gradient, from regions of lower concentration to higher concentration. To accomplish this, active transport proteins, known as ion pumps, utilize the energy released from ATP hydrolysis.
ATP-Driven Ion Pumps: The Workhorses of Active Transport
Ion pumps are embedded within cell membranes, operating as molecular gates that selectively allow the passage of specific ions. These pumps harness the energy from ATP hydrolysis to power the movement of ions across the membrane, establishing and maintaining concentration gradients.
Specific Ion Pumps: Maintaining Cellular Balance
A multitude of specific ion pumps exist, each meticulously tailored to transport particular ions. The sodium-potassium pump, for instance, plays a crucial role in maintaining the resting membrane potential of cells, while the calcium pump steadfastly regulates intracellular calcium levels.
ATP Hydrolysis: A Vital Force in Cellular Life
In conclusion, ATP hydrolysis provides the essential energy for active transport, enabling cells to maintain critical ion gradients. These gradients, in turn, underpin a vast array of physiological processes, including muscle contraction, nerve function, and nutrient absorption. Without ATP hydrolysis, the vital movement of ions across membranes would cease, and cellular life as we know it would be impossible.
Types of Ion Movement
In the realm of our cells, ions are constantly on the move, shaping the electrical and chemical balance that governs our very existence. These ions don’t travel haphazardly, but rather follow three distinct modes of movement: active transport, passive transport, and facilitated diffusion.
Active Transport: Pumping Against the Tide
Active transport is the muscular workhorse of ion movement, using energy from ATP hydrolysis to drive ions uphill against their concentration gradient. Like a water pump lifting water to a higher reservoir, active transport pumps ions against the flow of their own diffusion. This energy-demanding process is essential for maintaining the proper distribution of ions across cell membranes, such as the high potassium concentration inside cells and the low potassium concentration outside.
Passive Transport: Downhill and Effortless
Passive transport is a more relaxed mode of ion movement, taking advantage of the natural tendency of ions to flow down their concentration gradient. Like water flowing downhill, ions move from areas of high concentration to areas of low concentration, without the need for external energy input. This passive movement is essential for maintaining the equilibrium of ions across cell membranes.
Facilitated Diffusion: Smooth Sailing with Ion Channels
Facilitated diffusion, as the name suggests, provides an easier pathway for ions to cross cell membranes. Ion channels are specialized proteins that form pores in the membrane, allowing ions to pass through without having to penetrate the lipid bilayer. These channels can be gated, opening or closing in response to specific stimuli, or ungated, remaining permanently open. Facilitated diffusion is essential for rapid and selective ion movement, such as the movement of sodium and potassium ions during nerve transmission.
These three modes of ion movement work in concert to maintain the delicate balance of ions within and outside cells. Active transport pumps ions against their gradients, passive transport allows ions to flow down their gradients, and facilitated diffusion provides a speedy and selective pathway for ion movement. Together, they orchestrate the electrical and chemical symphony that drives our cells and ultimately our own bodies.