Unveiling The Elastic Rebound Hypothesis: Unraveling Earthquake Triggers And Rebound Effects
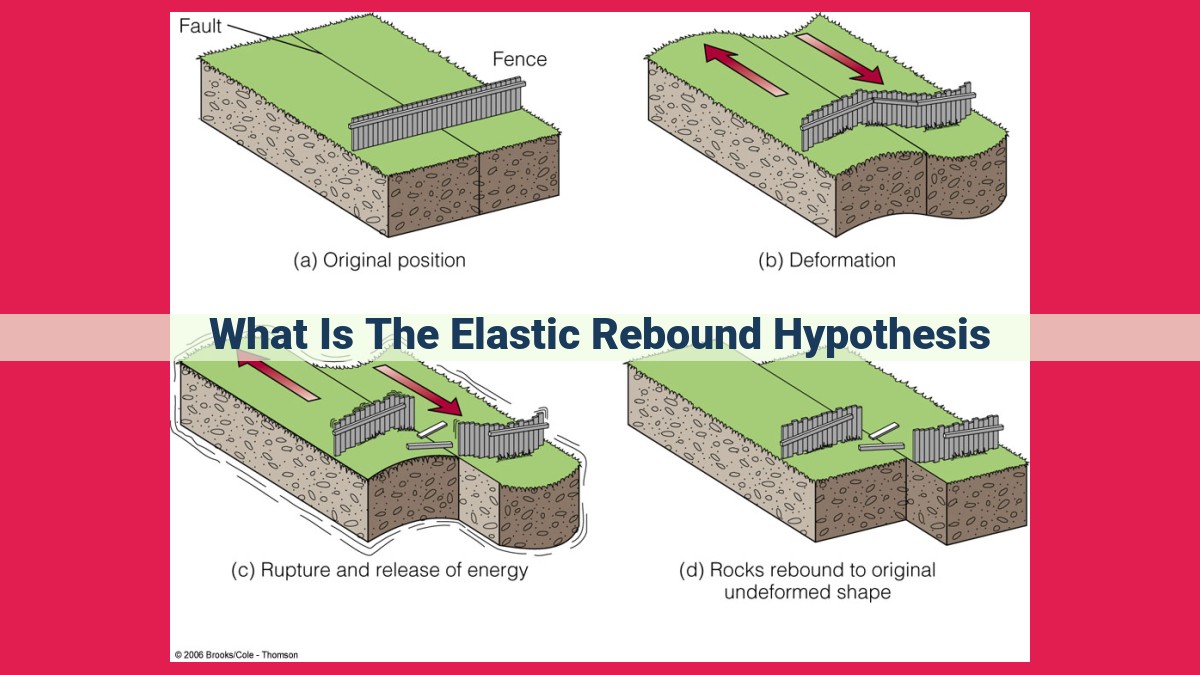
The Elastic Rebound Hypothesis suggests that earthquakes occur when tectonic plates gradually accumulate strain energy due to the buildup of stress on faults. When the stress overcomes the strength of the fault, it ruptures, releasing the accumulated energy as seismic waves. This process involves the elastic deformation of rocks, which return to their original shape after the earthquake, leading to the rebound effect. The hypothesis emphasizes the importance of strain, stress, and faults in understanding earthquake occurrence.
Understanding the Elastic Rebound Hypothesis: Unraveling the Forces Behind Earthquakes
Earthquakes, formidable forces of nature, have captivated scientists and intrigued humankind for centuries. Understanding the mechanisms that drive these seismic events is crucial for mitigating their devastating impacts. At the heart of earthquake science lies the Elastic Rebound Hypothesis, a pivotal theory that unveils the interplay of strain, stress, and faults in unleashing the Earth’s subterranean energy.
The Elastic Rebound Hypothesis proposes that earthquakes occur when accumulated strain within the Earth’s crust reaches a critical threshold, causing a sudden release of energy. Imagine a rubber band stretched beyond its elastic limit. When released, it snaps back to its original length, releasing the stored energy in the form of vibrations. Similarly, when rocks within the Earth’s crust are subjected to deformation (strain), they store energy. This energy accumulates over time until the rocks can no longer withstand the stress, triggering an earthquake.
Strain, a measure of deformation, occurs when rocks are stretched, compressed, or sheared. The amount of strain determines the magnitude of the earthquake. Stress, on the other hand, is the force per unit area that causes strain. The type of stress (tensional, compressive, or shear) influences the type of fault that forms and the direction of earthquake movement.
Faults, geological fractures in the Earth’s crust, serve as the boundaries where strain and stress concentrate. Different types of faults (strike-slip, reverse, normal) display distinct patterns of movement, contributing to the varied characteristics of earthquakes. The sudden release of strain along these faults generates seismic waves that travel through the Earth’s layers, causing the ground to shake.
Strain: The Buildup of Deformation
In the realm of earthquakes, strain plays a pivotal role in the buildup of energy that ultimately triggers these catastrophic events. Strain refers to the deformation or distortion of the Earth’s crust under the influence of forces.
There are three primary types of strain: tensile, compressive, and shear. Tensile strain occurs when the crust is stretched or pulled apart, causing it to elongate. Conversely, compressive strain results from forces that squeeze the crust, reducing its volume. Shear strain arises from forces that cause the crust to slide past one another, deforming its shape.
Within the Earth’s crust, strain accumulates gradually over time due to tectonic forces, such as the collision of plates or the movement of magma. As these forces persist, the crust undergoes deformation, building up potential energy.
The relationship between stress and strain is crucial in understanding earthquake dynamics. Stress refers to the force applied per unit area within the crust. When stress exceeds the strength of the crust, it fractures, releasing the accumulated energy in the form of an earthquake.
Strain and stress are inextricably linked. As strain increases, so does stress, until a critical point is reached where the crust can no longer withstand the forces acting upon it. This critical point is known as the yield point, beyond which the crust ruptures, triggering an earthquake.
By studying strain and stress in the Earth’s crust, scientists can gain insight into the buildup of energy and the likelihood of earthquakes occurring in specific regions. This knowledge helps them assess seismic hazards, develop early warning systems, and mitigate the potential impact of earthquakes on human populations.
Stress: The Forces at Play
- Define stress and explain different types of stress
- Discuss the forces involved in stress accumulation on faults and how they can trigger earthquakes
Stress: The Forces at Play
In the realm of earthquake science, understanding stress is crucial. Stress is the force per unit area acting on a material. On Earth’s crust, stress arises from various forces, including the gravitational pull of mountains, the collision of tectonic plates, and even the buoyancy of fluids within the crust.
Types of Stress
There are three main types of stress: tensional, compressional, and shear.
- Tensile stress occurs when a force pulls on an object, causing it to stretch or expand.
- Compressional stress occurs when a force pushes on an object, causing it to compress or shorten.
- Shear stress occurs when a force acts parallel to the surface of an object, causing it to slide or deform.
Stress Accumulation on Faults
Faults are fractures in the Earth’s crust where rocks have moved relative to each other. Stress can accumulate on faults over time when tectonic forces push or pull on the rocks on either side. When the stress exceeds the _strength of the rocks, the fault will slip and release energy in the form of an earthquake.
Forces Involved in Stress Accumulation
Several forces can contribute to stress accumulation on faults:
- Tectonic forces, such as plate collisions or spreading, can cause rocks on either side of a fault to move in opposite directions, generating shear stress.
- Gravitational forces, such as the weight of mountains, can cause rocks to compress, leading to compressive stress.
- Fluid pressure within the crust can also increase stress on faults, especially when fluids are trapped under impermeable layers.
Triggering Earthquakes
When stress on a fault exceeds the _strength of the rocks, the fault will rupture. The energy released during this sudden movement generates seismic waves that travel through the Earth’s crust and produce earthquakes. The amount of energy released and the magnitude of the earthquake depend on the size of the fault rupture and the amount of stress that has accumulated.
Faults: The Boundaries of Strain and Stress
In the intricate tapestry of the Earth’s crust, faults play a pivotal role in the dance of tectonic forces. Faults are fractures in the Earth’s crust where rocks have moved relative to each other. These geological boundaries mark the meeting point of strain and stress, where the Earth’s restless energy seeks release.
Faults come in various flavors, each with its unique story to tell. Dip-slip faults slide vertically, with one block moving up or down relative to the other. Strike-slip faults, on the other hand, slide horizontally, creating shearing forces that can cause significant ground motion.
The geometry of a fault, its inclination and orientation, influences the type of movement that can occur. Normal faults form when rocks pull apart, creating a step-like topography. Reverse faults, in contrast, occur when rocks push together, resulting in a thrust-like structure. Strike-slip faults slide laterally, producing a characteristic offset along the fault line.
The movement of faults is intimately connected to earthquake occurrence. When the accumulated strain on a fault exceeds the strength of the rocks, it can suddenly slip, releasing a burst of pent-up energy in the form of earthquake waves. The type of fault and its geometry influence the magnitude and intensity of the earthquake.
Dip-slip faults tend to generate earthquakes with more vertical ground motion, while strike-slip faults produce more horizontal shaking. The orientation of the fault relative to the populated areas determines the severity of the earthquake’s impact.
Understanding the characteristics and behavior of faults is crucial for earthquake preparedness and risk assessment. By studying the geometry and movement of faults, scientists can better predict the likelihood and potential impact of future earthquakes, helping us to mitigate their devastating consequences.
Epicenter: The Surface Impact
- Define the epicenter and explain its location relative to the earthquake source
- Discuss the relationship between the epicenter and ground motion
Epicenter: The Surface Impact of Earthquakes
Understanding the Epicenter
The epicenter is the point on the Earth’s surface directly above the focus, or the point where an earthquake originates deep beneath the crust. It marks the location where the seismic waves first reach the surface.
Relationship with Ground Motion
The epicenter plays a crucial role in understanding the impact of earthquakes on the surface. Ground motion, which includes shaking and vibrations, is strongest at the epicenter and decreases with distance from it. The intensity of ground motion at a particular location depends on factors such as the earthquake’s magnitude, depth, and the local geology.
Implications for Building Safety
Knowing the epicenter of an earthquake is vital for assessing its potential impact and implementing safety measures. By understanding the locations most likely to experience strong ground motion, communities can identify vulnerable structures and take steps to reinforce or relocate them. Proper building codes and construction practices can significantly reduce the risks associated with earthquakes at epicenters.
The epicenter is a critical aspect of earthquake understanding. It pinpoints the surface location where the shaking is most severe, helping us prepare for and mitigate the effects of these powerful geological events. By understanding the epicenter and its relationship with ground motion, we can safeguard our communities and make informed decisions about land use and building safety.
Focus: The Earthquake’s Origin
As the Earth trembles beneath our feet, it is crucial to determine the epicenter, the point on the surface directly above the earthquake source. But the true origin of an earthquake lies deeper, at its focus.
Defining the Focus
The focus, also known as the hypocenter, is the specific point within the Earth where the rupture or slippage along a fault initiates. It is located underground, and its depth provides vital information about the nature of the earthquake.
Location Relative to Epicenter and Fault
The focus is directly below the epicenter, which is the point on the Earth’s surface directly above it. The distance between the focus and the epicenter is known as the focal depth. The focal depth can vary significantly, ranging from a few kilometers to hundreds of kilometers.
Understanding Depth and Mechanism
The depth of the focus can shed light on the earthquake’s mechanism. Shallow earthquakes, with foci less than 70 kilometers deep, are typically caused by fault movement near the Earth’s surface. Intermediate-depth earthquakes, with foci between 70 and 300 kilometers, are often associated with subduction zones, where one tectonic plate slides beneath another. Deep earthquakes, with foci exceeding 300 kilometers, are rare and are believed to occur in the Earth’s mantle.
Furthermore, the focus helps scientists understand the earthquake’s mechanism. For example, strike-slip earthquakes occur when two tectonic plates slide past each other horizontally, while dip-slip earthquakes occur when two plates move vertically. By studying the focus, scientists can determine the type of fault movement that caused the earthquake.
In conclusion, the focus, the underground origin of an earthquake, provides valuable insights into its depth and mechanism. Understanding the focus helps scientists assess the potential impact of earthquakes and develop strategies for mitigation and preparedness.
Seismograph: Capturing the Earth’s Tremors
In the realm of earthquake science, the seismograph stands as an invaluable tool, a silent guardian tasked with capturing the Earth’s hidden tremors. This remarkable device, born from human ingenuity, has revolutionized our understanding of earthquakes and their enigmatic behavior.
Unveiling the Seismograph’s Mechanism
The secret behind the seismograph’s functionality lies in its ingenious design. At its core, a seismograph is a device that detects and records ground motion caused by earthquakes and other seismic events. Armed with sensitive sensors known as seismometers, these vigilant instruments meticulously translate the Earth’s subtle vibrations into electrical signals that can be analyzed by scientists.
A Symphony of Seismographs
The world of seismographs is a diverse one, with each type tailored to specific applications. Broadband seismographs excel in capturing a wide range of frequencies, from the gentle rumble of distant earthquakes to the high-pitched chirps of nearby tremors. In contrast, short-period seismographs focus on recording high-frequency vibrations, providing crucial insights into the immediate vicinity of an earthquake’s epicenter.
The Art of Earthquake Detection and Measurement
Seismographs not only detect earthquakes but also serve as precise instruments for measuring their magnitude and location. By analyzing the recorded waveforms, scientists can determine the amplitude of the seismic waves, a key indicator of an earthquake’s strength. The arrival times of these waves provide invaluable information about the earthquake’s epicenter and depth.
A Global Network of Seismic Sentinels
Across the globe, a vast network of seismographs stands vigil, forming a dedicated army of silent observers. These tireless sentinels tirelessly monitor the Earth’s seismic activity, providing scientists with a comprehensive picture of earthquake patterns and risks worldwide. By combining data from multiple seismographs, experts can pinpoint the exact location of an earthquake, assess its magnitude, and even estimate its potential impact.
Unveiling the Hidden World of Earthquakes
The seismograph has empowered scientists to venture into the enigmatic world of earthquakes, unveiling secrets that were once shrouded in darkness. Through the lens of these instruments, we have gained unprecedented insights into the Earth’s internal dynamics, the forces that shape our planet, and the risks that lurk beneath our feet.