Dna Structure: Unveiling The Enigma Of The Double Helix And Its Variations
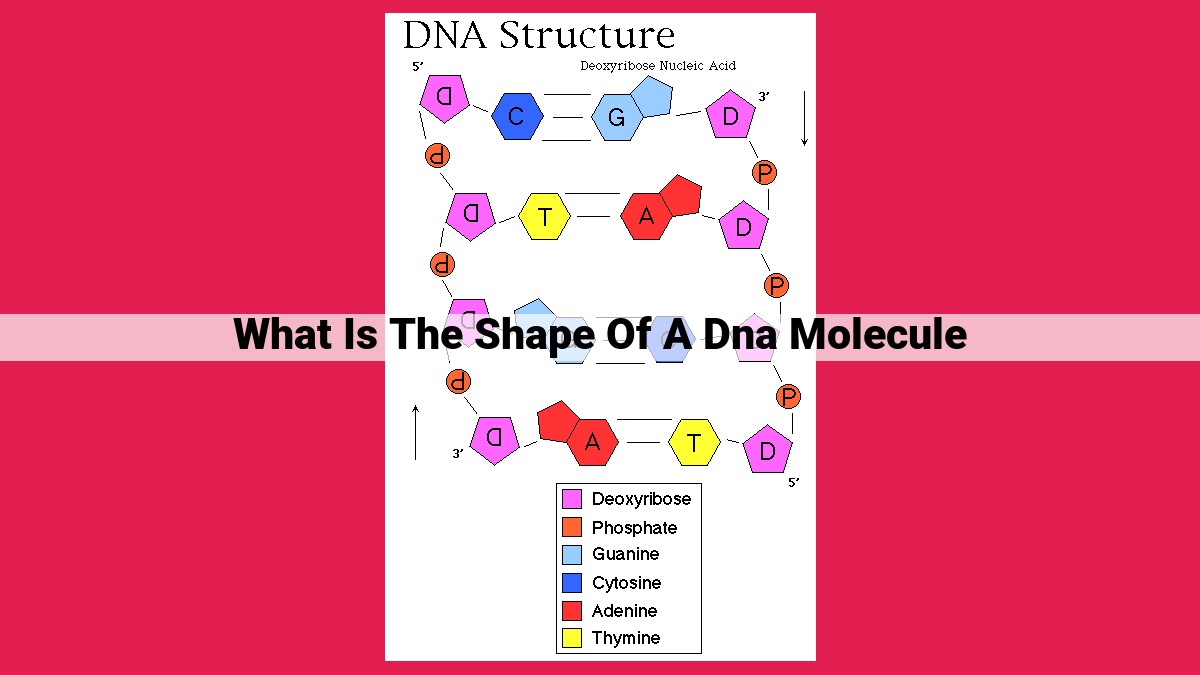
DNA’s iconic double helix shape is formed by two antiparallel strands connected by hydrogen-bonded base pairs (A-T, G-C). This ladder-like structure features major and minor grooves, providing sites for recognition and binding. Variations in DNA shape, including B-form (standard), A-form (narrower grooves), and Z-form (left-handed twist), arise from different base compositions and environmental factors. Additionally, DNA can undergo supercoiling, a dynamic twisting that affects its structure and biological functions, such as packaging and gene regulation.
The Double Helix: DNA’s Structural Foundation
- Describe the two antiparallel strands, their 5′ and 3′ orientations, and how they form a ladder-like structure.
- Explain the role of hydrogen bonds between complementary base pairs (A-T, G-C) in maintaining strand cohesion.
- Discuss the significance of the major and minor grooves, and their relevance to DNA recognition and binding.
- Highlight the characteristic right-handed twist of the DNA double helix.
Unraveling DNA’s Structural Symphony: The Double Helix Foundation
At the heart of life’s blueprint lies a remarkable molecule: DNA. Its intricate structure, shaped as an iconic double helix, serves as the cornerstone of our genetic inheritance.
The Ladder of Life: Antiparallel Strands Align
DNA’s structure resembles a twisted ladder, consisting of two antiparallel strands. Antiparallel means these strands run in opposite directions, with each strand having a 5′ phosphate group at one end and a 3′ hydroxyl group at the other.
Hydrogen Bonds: The Sticky Zipper
The two strands of DNA are held together by a series of hydrogen bonds. These bonds form between complementary base pairs: adenine (A) pairs with thymine (T), and guanine (G) pairs with cytosine (C). These pairings create a ladder-like structure with alternating rungs and rails.
The Grooves: A Tale of Two Depths
The double helix has two distinct grooves: the major groove and the minor groove. The major groove is wider and shallower than the minor groove, providing a platform for proteins and other molecules to bind to DNA and interact with its genetic information.
The Right-Handed Twist: DNA’s Signature Curve
DNA’s double helix exhibits a distinctive right-handed twist, resembling a spiral staircase. This unique twist allows DNA to fit snugly within the confines of cells, enabling it to carry and transmit genetic information efficiently.
Variations in DNA Shape: Beyond the Double Helix
- Introduce the three main forms of DNA: B-form, A-form, and Z-form.
- Describe the structural differences between these forms, including their groove widths and helical parameters.
- Explain the factors that influence DNA shape, such as base composition, hydration level, and protein binding.
Variations in the Shape of DNA: Beyond the Double Helix
While we’re familiar with the iconic double helix structure of DNA, it’s not the only form this remarkable molecule can take. In fact, DNA can exhibit a variety of shapes, each with its own unique characteristics and biological significance.
Meet the DNA Family: A-Form, B-Form, and Z-Form
Just like there are different shapes of pasta, DNA also comes in different configurations. The three main forms are the A-form, B-form, and Z-form.
- A-form: This compact form is found in conditions of low hydration and when DNA contains a high proportion of adenine (A) and thymine (T) bases. It has a right-handed double helix but a wider and shallower major groove compared to the B-form.
- B-form: This is the most common form of DNA under physiological conditions, with a right-handed double helix characterized by a narrower and deeper major groove.
- Z-form: This unique form is characterized by a left-handed double helix and a zigzag pattern. It’s found in certain regions of DNA where there’s a high proportion of guanine (G) and cytosine (C) bases.
Factors Shaping DNA’s Dance
The shape of DNA is not static, but rather influenced by several factors:
- Base composition: Each base pair, whether it’s A-T or G-C, has a slightly different size and shape, affecting the overall geometry of the helix.
- Hydration level: The amount of water surrounding DNA can cause it to switch between A-form and B-form.
- Protein binding: Certain proteins that interact with DNA can induce changes in its shape, influencing gene regulation and other biological processes.
The Significance of Shape
The different shapes of DNA are not merely aesthetic variations. They play crucial roles in DNA’s biological functions:
- Gene storage and transmission: The double helix structure allows for the secure storage and accurate transmission of genetic information.
- DNA binding proteins and transcription factors: Specific proteins recognize and bind to specific DNA shapes, regulating gene expression and controlling cellular processes.
- DNA replication and repair: The ability of DNA to adopt different shapes is essential for replication and repair mechanisms to function efficiently.
In summary, DNA’s shape is a dynamic and multifaceted aspect of its structure, with significance for various biological processes. Understanding the variations in DNA shape not only enhances our knowledge of its fundamental properties but also provides insights into the intricate workings of the cell.
Supercoiling: DNA’s Dynamic Twist
Supercoiling is a phenomenon that occurs when DNA is twisted upon itself, creating a dynamic and intricate structure. This twisting can be either positive or negative, depending on the direction of the coils.
Positive supercoiling arises when the DNA is twisted in a right-handed direction, resulting in a more compact structure. Conversely, negative supercoiling occurs when the DNA is twisted left-handed, creating a more extended and relaxed configuration.
Topoisomerases are essential enzymes that manage supercoiling and maintain DNA stability. These enzymes can selectively break and rejoin DNA strands, allowing them to relieve or introduce supercoils as needed.
Supercoiling plays a crucial role in various biological processes:
- DNA Packaging: Supercoiling helps package DNA into the compact structures found in cells, such as chromosomes and nucleosomes.
- Gene Regulation: Supercoiling can influence gene expression by altering the accessibility of DNA to transcription factors and other regulatory proteins.
- DNA Replication: Supercoiling can facilitate DNA replication by providing the necessary tension to unwind and separate the DNA strands.
Understanding the intricacies of supercoiling is essential for unraveling the mysteries of DNA’s dynamic nature and its profound impact_ on cellular processes.
The Dynamic Shape of DNA: A Blueprint for Life
DNA, the molecule that holds the blueprints for life, is not a static entity. It is a dynamic structure whose shape plays a crucial role in its biological functions. From the iconic double helix to its variations and dynamic twists, DNA’s shape dictates its interactions with proteins, enzymes, and cellular machinery.
The Double Helix: DNA’s Foundation
The renowned double helix of DNA consists of two antiparallel strands twisted around each other. The complementary base pairs (A-T and G-C) form a ladder-like structure, held together by hydrogen bonds. The major and minor grooves on the surface of the helix serve as recognition points for proteins and other molecules.
Variations in DNA Shape
DNA is not confined to the double helix. It can also adopt other forms, such as the A-form and Z-form. These variations in shape arise from differences in base composition, hydration level, and protein binding. Understanding these variations is essential for comprehending DNA’s interaction with various cellular components.
Supercoiling: Twisting and Unwinding DNA
DNA is not a rigid molecule. It can undergo supercoiling, a process that introduces positive or negative twists into the double helix. Supercoiling is crucial for DNA packaging, gene regulation, and replication. Enzymes known as topoisomerases manage supercoiling, ensuring DNA stability and proper functioning.
The Importance of DNA Shape
DNA’s shape is fundamental to its biological roles:
-
Gene Storage and Transmission: The double helix stores genetic information securely, allowing for faithful transmission of genetic instructions to daughter cells during cell division.
-
DNA Binding Proteins and Transcription Factors: The grooves of DNA provide binding sites for proteins that regulate gene expression by interacting with specific DNA sequences.
-
DNA Replication and Repair: The dynamic shape of DNA allows it to undergo unwinding and rewinding during replication and repair processes, ensuring the accurate duplication and restoration of genetic material.