Unveiling The Mechanisms Of Dna Replication: Dna Unwinding And The Importance Of Enzyme Activity
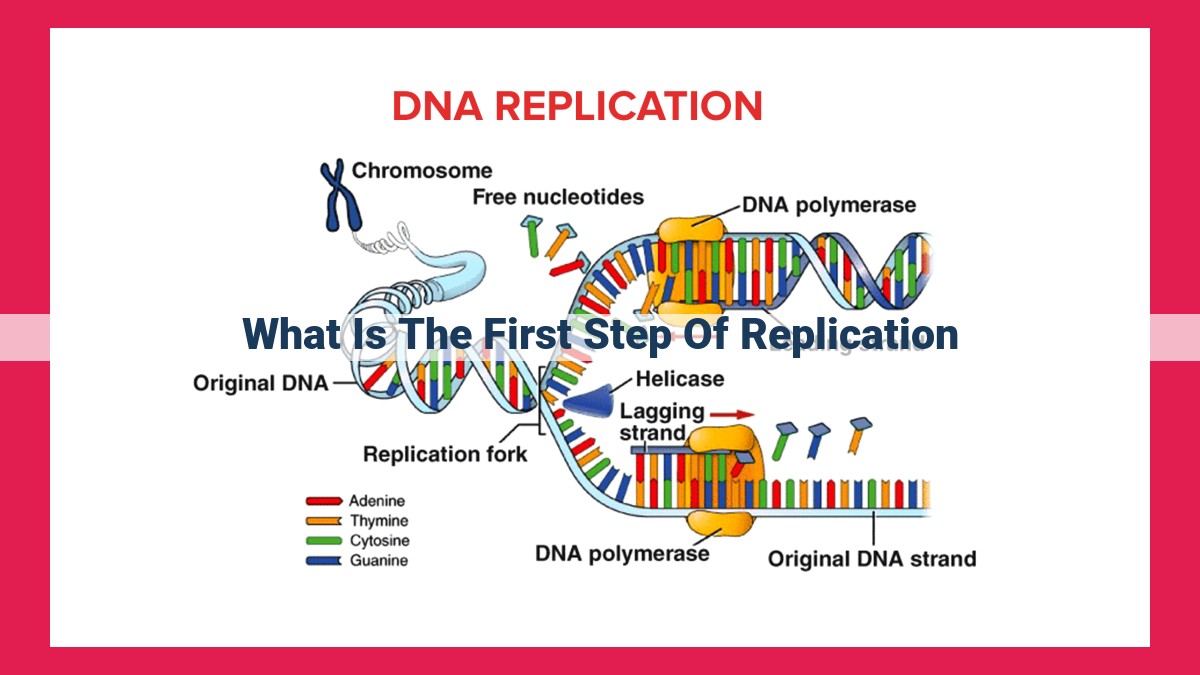
DNA replication initiates with DNA unwinding. DNA helicase, an enzyme, breaks the hydrogen bonds between complementary DNA strands, separating them into single strands. Single-stranded binding proteins stabilize the separated strands, preventing them from re-annealing. The unwound region forms a replication bubble, the designated zone for DNA replication. This step is crucial as it creates the template for DNA polymerase to synthesize new strands, ensuring faithful duplication of genetic information.
DNA Replication: An Overview
- Importance and basic steps of DNA replication
DNA Replication: An Overview
DNA replication is a critical process that ensures the accurate transmission of genetic information during cell division. It plays a pivotal role in the inheritance of traits, the growth and development of organisms, and the maintenance of genetic stability.
The basic steps of DNA replication involve:
- DNA unwinding: The double-stranded DNA unwinds, separating the two strands to create a replication bubble.
- Single-stranded binding proteins: These proteins bind to the separated strands, preventing them from re-annealing.
- Leading strand synthesis: One strand, known as the leading strand, is synthesized continuously in the 5′ to 3′ direction.
- Lagging strand synthesis: The other strand, called the lagging strand, is synthesized discontinuously in short fragments called Okazaki fragments. These fragments are later joined together.
These processes are essential for the accurate duplication of genetic material, ensuring the preservation of cell identity and the continuity of life.
DNA Unwinding: Separating the Strands
In the intricate process of DNA replication, a crucial step lies in the separation of the DNA strands. Just as a zipper needs to be unzipped before it can be replaced, the tightly coiled DNA double helix must be unwound to allow access for the machinery that will create new strands.
This intricate task falls upon a molecular maestro known as DNA helicase. Picture a tiny pair of molecular scissors, gliding along the DNA double helix and snipping the hydrogen bonds that hold the two strands together. As DNA helicase works its magic, the DNA strands begin to separate, forming a Y-shaped region known as the replication fork.
The unwinding of DNA is not a passive process. DNA helicase requires energy in the form of ATP to fuel its unwinding machinery. Moreover, to prevent the strands from re-annealing and disrupting the replication process, single-stranded binding proteins (SSBs) step in. These proteins bind to the exposed single strands, acting as molecular chaperones that keep them separated and ready for replication.
Thus, the unwinding of DNA is a critical and carefully orchestrated process, ensuring that the genetic blueprint can be accurately copied and passed on to future generations.
Single-Stranded Binding Proteins: Guardians of DNA Replication
In the intricate world of DNA replication, single-stranded binding proteins (SSBs) emerge as unsung heroes. Their critical mission is to prevent the unwound DNA strands from re-annealing, ensuring the smooth and accurate duplication of genetic information.
As DNA helicase works tirelessly to unzip the DNA double helix, creating a replication bubble, SSBs step into action. These proteins bind tightly to the exposed single-stranded DNA, like tiny chaperones, keeping them separated and accessible for the replication machinery.
The importance of SSBs cannot be overstated. Without their intervention, the unwound DNA strands would quickly snap back together, hindering the progression of replication. Their presence stabilizes the single-stranded state, creating a crucial window of opportunity for the replication enzymes to work their magic.
Moreover, SSBs play a vital role in the recruitment of other replication factors to the site of replication. By maintaining the single-stranded DNA structure, they facilitate the binding of polymerases and accessory proteins, ensuring the efficient and precise assembly of new DNA molecules.
In essence, SSBs act as guardians of DNA replication, ensuring that the genetic code is copied faithfully and without interruption. Their unassuming yet essential presence safeguards the integrity of our genetic heritage, laying the foundation for the continuity of life itself.
The Birthplace of New DNA: Unraveling the DNA Replication Bubble
DNA replication, the intricate process of creating an identical copy of genetic material, occurs within a specialized structure known as the DNA replication bubble. Imagine a tiny, bustling construction site where DNA molecules are meticulously unzipped and duplicated with astonishing precision.
At the heart of this bubble lies the origin of replication, a designated starting point where the DNA double helix begins to unwind like a zipper. As the DNA strands separate, a bubble-like structure forms, providing ample space for the replication machinery to operate.
To ensure that the separated strands remain unraveled and accessible for copying, a team of essential proteins steps into action. DNA helicase, a molecular powerhouse, cleaves the hydrogen bonds holding the DNA bases together, effectively unzipping the double helix. Simultaneously, single-stranded binding proteins coat the exposed DNA strands, preventing them from reattaching prematurely and disrupting the replication process.
With the DNA strands separated, the stage is set for the synthesis of new DNA molecules. On one side, the leading strand is synthesized continuously, as the DNA polymerase enzyme effortlessly extends the growing DNA strand in the direction of the replication fork.
On the opposite side, the lagging strand faces a more complex challenge. Due to the antiparallel nature of DNA strands, it must be synthesized in small fragments known as Okazaki fragments. These fragments are subsequently stitched together by DNA ligase, a molecular glue that seamlessly joins the pieces, creating the continuous lagging strand.
The DNA replication bubble is a dynamic and highly orchestrated microcosm, where the delicate balance of unwinding, binding, and synthesis ensures the faithful replication of genetic material. As the replication bubble expands, new copies of DNA emerge, carrying the genetic blueprints that drive life and evolution.
The Leading Strand: A Smooth and Continuous Symphony
In the intricate dance of DNA replication, a delicate balance unfolds between the continuous and discontinuous synthesis of new strands. The leading strand, a veritable storyteller, embarks on a seamless journey, unfurling its genetic narrative with an uninterrupted flow of nucleotides.
The DNA helicase, the master of unwinding, orchestrates the separation of the DNA double helix, creating a replication bubble where the replication machinery takes center stage. As the leading strand elongates, a DNA polymerase, a skilled scribe, deftly adds nucleotides one by one, guided by the existing template strand. This process, known as continuous synthesis, mimics the rhythm of a flowing river, where nucleotides are seamlessly incorporated into the growing strand.
The leading strand’s uninterrupted progress is a marvel of molecular efficiency, ensuring the preservation of genetic information without the need for complex assembly or repair. It stands as a testament to the intricate elegance and precision of the cellular machinery responsible for life’s most fundamental process.
Lagging Strand: The Discontinuous Synthesis
As the DNA replication bubble expands, the formation of two new strands begins. One strand, known as the leading strand, synthesizes continuously in the 5′ to 3′ direction. However, the other strand, the lagging strand, encounters a unique challenge.
Unlike the leading strand, the lagging strand cannot be synthesized continuously due to the antiparallel orientation of the DNA molecule. Instead, it is synthesized discontinuously in short fragments called Okazaki fragments.
The process of lagging strand synthesis involves several steps:
-
Primase, an enzyme, synthesizes an RNA primer, a short piece of RNA complementary to the template strand. This primer provides a starting point for DNA polymerase.
-
DNA polymerase III, the main enzyme responsible for DNA synthesis, elongates the RNA primer by adding deoxynucleotides. It synthesizes the Okazaki fragments in the 5′ to 3′ direction.
-
As the lagging strand is synthesized discontinuously, the Okazaki fragments need to be joined together. This task is performed by DNA ligase, an enzyme that covalently bonds the fragments.
The process of Okazaki fragment assembly is crucial for lagging strand synthesis. These fragments ensure that the entire template strand is copied accurately, allowing for the transmission of complete genetic information to the daughter cells.
Primase: The Catalyst for DNA Synthesis
Imagine DNA replication as a meticulous construction project, where an identical copy of the original blueprint must be meticulously crafted. At the heart of this process lies primase, the enzyme that initiates DNA synthesis, acting as the architect’s first brushstroke on the canvas of genetic material.
Primase, the forefather of DNA synthesis, plays a crucial role in the intricate dance of replication. It recognizes and binds to single-stranded DNA, the template for the new strand, and initiates the process of synthesis. Unlike the polymerase enzymes that elongate the DNA strand, primase’s modest repertoire includes the ability to create short RNA segments called primers. These primers provide a crucial starting point for DNA polymerase, which can only add nucleotides to an existing strand.
The delicate task of primer synthesis requires primase to thread RNA nucleotides into place, momentarily replacing DNA polymerase. However, primase’s work is temporary, as DNA polymerase eventually displaces the RNA primers and replaces them with DNA nucleotides. This intricate interplay between primase and DNA polymerase ensures the seamless and accurate duplication of genetic information.
Okazaki Fragments: Building Blocks of the Lagging Strand
- Role of Okazaki fragments in discontinuous synthesis
Okazaki Fragments: The Building Blocks of the Lagging Strand
The process of DNA replication involves the precise copying of genetic information from one generation of cells to the next. It’s a complex endeavor, and one of the challenges is that DNA, the double-stranded molecule that carries our genetic code, has to be unwound and separated during replication.
In most cases, DNA replication proceeds in the 5′ to 3′ direction, meaning that the newly synthesized DNA strand grows from the 5′ end towards the 3′ end. This process is known as leading strand synthesis and is continuous, as the DNA polymerase enzyme can add nucleotides to the growing strand without interruption.
However, one of the strands of DNA, the lagging strand, is synthesized in a discontinuous manner. This is because the DNA polymerase enzyme can only add nucleotides to the 3′ end of a growing strand, and since the lagging strand is oriented in the opposite direction, the enzyme has to work backwards.
To overcome this challenge, DNA polymerase synthesizes short fragments of DNA, known as Okazaki fragments, in the 5′ to 3′ direction. These fragments are then joined together by another enzyme, DNA ligase, to form a continuous strand.
The formation of Okazaki fragments is a crucial step in DNA replication, ensuring that both strands of the DNA molecule are accurately copied and that the genetic information is preserved. These fragments are named after _Reiji Okazaki, a Japanese biochemist who discovered them in the 1960s.
Understanding the role of Okazaki fragments in DNA replication is essential for comprehending how cells maintain their genetic integrity and pass on genetic information to future generations.
Origin of Replication: The Starting Point
The journey of DNA replication commences at a specific location on the DNA molecule, known as the origin of replication. This region serves as the starting point for the unwinding and copying of the genetic material.
The origin of replication is characterized by its unique nucleotide sequence, which is recognized by specific proteins called initiation factors. These proteins bind to the origin and facilitate the unwinding of the DNA double helix, allowing access to the genetic code for replication.
Each chromosome possesses multiple origins of replication, ensuring that DNA replication can occur simultaneously at multiple locations. This multi-origin mechanism expedites the duplication process, allowing for the efficient transmission of genetic information to daughter cells during cell division.