Dna Replication: A Comprehensive Guide To The Process Of Duplicating Genetic Material
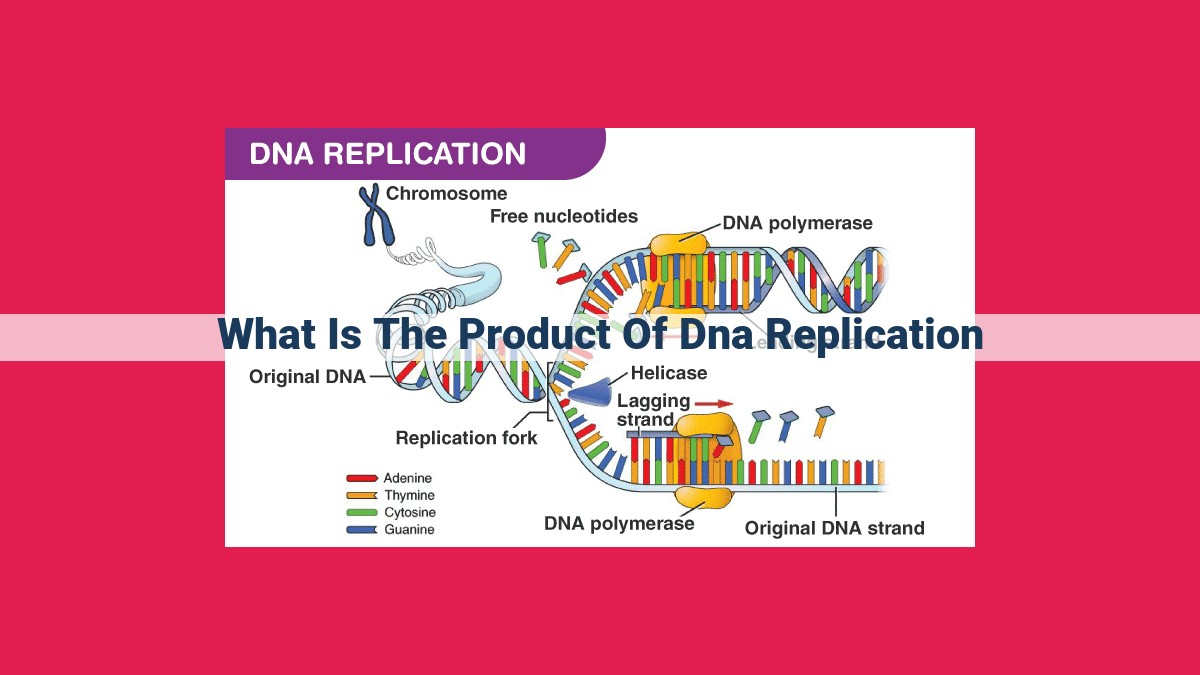
The product of DNA replication is two identical new DNA molecules, each made up of one parental strand and one newly synthesized strand. This process, known as semi-conservative replication, occurs through the coordinated action of several enzymes and proteins. Key components include the replication fork, leading and lagging strands, Okazaki fragments, and enzymes such as helicase, primase, and DNA polymerase, among others. Together, these components facilitate the unwinding, priming, and synthesis of the new DNA strands, ultimately resulting in the duplication of the genetic material.
DNA Replication: The Blueprint of Life
In the intricate tapestry of life, DNA (deoxyribonucleic acid) reigns supreme as the blueprint that guides all living organisms. Within each cell, this molecule holds the genetic instructions that determine our traits, characteristics, and even our destiny. To ensure the faithful transmission of this precious information, DNA must undergo a meticulous copying process known as replication.
At the heart of DNA replication lies the daughter DNA molecules. These are the end products of the process, each carrying an exact copy of the original DNA. The remarkable fidelity of DNA replication guarantees the accurate inheritance of genetic information from parent cells to their progeny.
The machinery responsible for DNA replication is a complex orchestra of proteins, each playing a vital role. Together, they unweave the double helix, synthesize new strands, and meticulously join them, ensuring the seamless duplications of our genetic blueprint. Join us as we delve into the captivating world of DNA replication, deciphering the intricacies of this fundamental process that underpins the very fabric of life.
DNA Replication: The Tale of Two Identical Daughters
In the bustling metropolis of the cell, there unfolds a remarkable tale of replication, a process so crucial that it ensures the seamless transfer of genetic information from one generation to the next. At the heart of this story lies the creation of two identical daughter DNA molecules, the end products of a meticulously orchestrated dance known as semi-conservative DNA replication.
Semi-conservative means that each daughter molecule inherits one of the two original DNA strands, while the other new strand is synthesized to complement it. This process is carried out by an intricate ensemble of molecular players, each with a specific role to orchestrate the precise duplication of DNA.
At the replication fork, the site of this intricate process, the DNA double helix unwinds like a zipper. Helicase, the master unwinder, relentlessly breaks the hydrogen bonds between DNA nucleotides, exposing single strands. Single-stranded binding proteins (SSBs), like protective chaperones, immediately rush to stabilize these vulnerable strands.
As the DNA helix gracefully unwinds, primase, the priming enzyme, steps into action. Its nimble fingers synthesize short RNA primers, essential starting points for the next star of our tale, DNA polymerase. This molecular maestro meticulously adds nucleotides to the growing DNA strands, using the existing strands as templates.
But hold on a moment! DNA replication isn’t always a smooth, continuous process. On one strand, known as the leading strand, DNA polymerase can merrily synthesize continuously in the direction of the unwinding fork. However, on the other strand, the lagging strand, things get a bit more fragmented.
Okazaki fragments, small pieces of DNA, are synthesized discontinuously on the lagging strand. Primase once again plays a vital role, starting each new fragment with a fresh primer. DNA ligase, the final craftsman in our tale, joins these fragments together into a continuous strand, completing the intricate tapestry of DNA replication.
The Replication Fork: The Hub of DNA Replication
DNA replication is a fundamental process in biology, ensuring the accurate transmission of genetic information from one generation to the next. At the heart of this intricate dance lies the replication fork, a dynamic structure that represents the site of active DNA replication.
Imagine the DNA double helix as a tightly coiled zipper. To replicate this genetic blueprint, the zipper must be painstakingly unzipped and copied, creating two identical daughter molecules. This is where the replication fork enters the picture.
The replication fork is a Y-shaped structure, where the two strands of DNA separate, exposing the base pairs that serve as the template for new strand synthesis. Helicase, the molecular “unwinding machine,” breaks the hydrogen bonds between the base pairs, while primase lays down short RNA primers that guide the DNA polymerases, the molecular “copying machines,” to begin adding nucleotides to the growing daughter strands.
Crucially, single-stranded binding proteins (SSBs) keep the unwound DNA single-stranded, preventing it from re-annealing and disrupting the replication process. Topoisomerase plays a supporting role, relieving tension in the DNA double helix as it unwinds.
The replication fork is a bustling hub of activity, with multiple molecules working in concert to ensure the accurate duplication of DNA. The intricate interplay of helicase, primase, SSBs, topoisomerase, and DNA polymerases is a testament to the remarkable precision of biological processes.
Leading and Lagging Strands: The Dance of DNA Replication
As the DNA replication machinery unfolds, it encounters a fascinating tale of two strands: the leading strand and the lagging strand. Imagine a高速公路, where two cars race side-by-side, representing the replication forks on each strand.
The leading strand has it easy. Like a car in the fast lane, it races ahead, continuously adding nucleotides to its growing chain. But the lagging strand faces a challenge. It’s like a car stuck in the slow lane, constantly lagging behind its counterpart.
To overcome this obstacle, the lagging strand employs a clever strategy. It synthesizes Okazaki fragments, short segments of DNA that are assembled into a continuous strand later on. These fragments are like puzzle pieces, each one fitting together to form the lagging strand.
Imagine a team of workers, each responsible for building a specific puzzle piece. As the replication fork moves along the lagging strand, these workers (known as DNA polymerase III) lay down the nucleotides for each Okazaki fragment. But how do they know where to start?
Enter primase, the “signaling” enzyme. It marks the starting point for each Okazaki fragment by synthesizing a short RNA primer. Once the primer is in place, DNA polymerase III can get to work, adding nucleotides until it reaches the end of the fragment.
As each Okazaki fragment is completed, it’s handed off to DNA ligase, the “construction crew.” DNA ligase seamlessly joins the fragments together, creating a continuous lagging strand that matches its leading strand counterpart.
And so, the dance of DNA replication continues, with the leading strand gliding ahead and the lagging strand catching up through its intricate assembly of Okazaki fragments. This intricate process ensures that each daughter DNA molecule is a perfect copy of the original, preserving the genetic information that defines life on Earth.
Okazaki Fragments: The Building Blocks of the Lagging Strand
As we delve deeper into the fascinating process of DNA replication, we encounter the intricate mechanism that ensures the faithful duplication of our genetic blueprint. At the heart of this process lies the replication fork, where the DNA double helix unwinds, revealing single strands that serve as templates for the synthesis of new DNA molecules.
One of the key players in this replication dance is the lagging strand, which faces a unique challenge. Unlike its leading strand counterpart, which is synthesized continuously, the lagging strand is built in fragments known as Okazaki fragments. These fragments are named after their discoverer, Reiji Okazaki, and play a vital role in ensuring the accuracy and efficiency of DNA replication.
Formation and Synthesis of Okazaki Fragments
Okazaki fragments arise from the unique structure of DNA polymerase, the enzyme responsible for adding nucleotides to the growing DNA strand. DNA polymerase can only extend a DNA strand in the 5′ to 3′ direction, which means that it can only add nucleotides to the 3′ end of an existing strand.
On the leading strand, DNA polymerase can continuously add nucleotides to the 3′ end as the replication fork unwinds the DNA helix. However, on the lagging strand, the unwinding process creates a single-stranded DNA template that is moving in the opposite direction. To overcome this obstacle, primase, another key enzyme, steps in to synthesize short RNA primers that provide a starting point for DNA polymerase on the lagging strand.
Primase and the Initiation of Okazaki Fragments
Primase is a special type of RNA polymerase that synthesizes short RNA segments called primers. These primers are typically around 10 nucleotides long and provide a free 3′ end for DNA polymerase to attach to and begin extending the DNA strand.
Once a primer is in place, DNA polymerase can bind and begin adding nucleotides to the 3′ end of the primer, extending the DNA strand in the 5′ to 3′ direction. However, once the DNA polymerase reaches the end of the primer, it falls off, leaving a gap in the DNA strand.
To fill in these gaps, DNA polymerase III, the main enzyme responsible for DNA synthesis, synthesizes new Okazaki fragments. These fragments are typically around 100 to 200 nucleotides long and are synthesized in the 5′ to 3′ direction, overlapping with the previous fragment.
Okazaki Fragments and Lagging Strand Synthesis
The continuous synthesis of Okazaki fragments on the lagging strand is a remarkable feat of coordination and teamwork among the replication machinery. Primase ensures that primers are available for DNA polymerase to initiate Okazaki fragments, while DNA polymerase III efficiently extends these fragments, filling in the gaps to create a continuous DNA strand.
Once all the Okazaki fragments have been synthesized, they are joined together by another enzyme, DNA ligase, which seals the gaps, creating a continuous DNA molecule on the lagging strand. This process of Okazaki fragment synthesis and ligation is a critical step in DNA replication, ensuring that both the leading and lagging strands are accurately and completely duplicated.
DNA Polymerase: The Maestro of DNA Replication
In the intricate world of DNA replication, where genetic blueprints are meticulously copied, DNA polymerase stands as the master craftsman, adding nucleotides one by one to the growing DNA strands.
Just as a painter uses his brush to create a masterpiece, DNA polymerase, with its exquisite precision, paints the genetic canvas, meticulously adding nucleotides to form the double helix. But this extraordinary enzyme does not work in isolation. It relies on two key helpers: primase and helicase.
Primase, like an artist sketching the initial outlines, lays down short RNA primers, providing a foundation for DNA polymerase to build upon. These primers act as starting points, guiding DNA polymerase as it adds nucleotides in a 5′ to 3′ direction, elongating the DNA strand.
Helicase, on the other hand, plays the role of an unsung hero, diligently unwinding the DNA double helix, creating the replication fork where DNA polymerase can work its magic. This unwinding process is essential, allowing the enzyme access to the DNA template.
Together, primase, helicase, and DNA polymerase form an unstoppable trio, collaborating to ensure the accurate and efficient duplication of genetic material. Without these key players, DNA replication would falter, leading to genetic chaos.
So, as you marvel at the intricate beauty of life, remember the extraordinary tale of DNA polymerase, the enzyme that, with its partners in crime, ensures the continuity of life’s grand design.
Primase: The Unsung Wizard Behind DNA Replication
In the intricate world of DNA replication, an often-overlooked enzyme plays a crucial role in kickstarting the process – Primase, the priming enzyme. Without primase, our cells would be unable to faithfully copy their genetic blueprint, leading to potential chaos and errors.
Primase is the maestro that initiates the DNA replication process by synthesizing short RNA primers. These primers are essential for DNA polymerases, the enzymes that add nucleotides to the growing DNA strands, to begin their work. Without these RNA primers, DNA polymerases would be unable to add nucleotides to the growing DNA strands, and DNA replication would come to a grinding halt.
The importance of primase cannot be overstated. It ensures that DNA replication occurs with accuracy and precision, preserving the integrity of our genetic material.
Additional Information:
-
Primase is an RNA polymerase that uses ribonucleotides as building blocks.
-
The primers synthesized by primase are typically around 10-12 nucleotides long.
-
Primase is essential for both leading and lagging strand synthesis.
-
The leading strand is synthesized continuously, while the lagging strand is synthesized in fragments called Okazaki fragments that are later joined together by DNA ligase.
Helicase: Unwinding the Double Helix for DNA Replication
In the intricate dance of DNA replication, a vital enzyme known as helicase takes center stage. Its mission: to unravel the tightly wound DNA double helix, creating a pathway for the replication machinery to access and duplicate each nucleotide sequence.
Breaking the Hydrogen Bond Bonds of Life
Imagine DNA as a twisted ladder. Each rung of the ladder consists of a pair of nucleotides, held together by hydrogen bonds. Helicase acts like a molecular key, breaking these hydrogen bonds and uncoiling the DNA strands into two separate strands. This unwinding process creates a “replication fork,” a Y-shaped region where DNA replication initiates.
Unwinding with Assistance
Helicase does not work alone. It relies on two support crew: SSBs (Single-Stranded Binding Proteins) and topoisomerase. SSB proteins stabilize the unwound DNA strands, preventing them from reannealing. Topoisomerase, on the other hand, relieves tension that would otherwise build up in the DNA helix due to unwinding.
Unveiling the Hidden Code
The unwound DNA strands now lie exposed, ready to be read and copied by other enzymes. This process is essential for DNA replication, the process by which cells create identical copies of their genetic material. By unwinding the DNA helix, helicase ensures that the replication machinery has unobstructed access to the genetic code, allowing for the faithful transmission of genetic information from one generation of cells to the next.
In Summary:
- Helicase is an enzyme that unravels the DNA double helix.
- It breaks hydrogen bonds between nucleotides.
- SSBs and topoisomerase assist helicase in its unwinding function.
- Unwinding DNA is crucial for DNA replication, as it allows for access to the genetic code.
Single-Stranded Binding Proteins (SSBs): Guardians of Unraveled DNA
As DNA helicase diligently unwinds the double helix during replication, it exposes fragile single-stranded DNA. Enter single-stranded binding proteins (SSBs), the unsung heroes that safeguard these vulnerable strands.
SSBs are like tiny molecular chaperones that bind and stabilize single-stranded DNA. Think of them as guardians who prevent the exposed strands from re-annealing prematurely or forming tangles known as hairpins.
Their stabilizing grip allows helicase to continue unwinding the helix. Without SSBs, helicase would have a much harder time performing its critical task, making DNA replication less efficient and accurate.
Topoisomerase: Relieving Tension in the DNA Helix:
- Explain the function of topoisomerase in relieving tension and supercoiling in the DNA helix.
- Describe how topoisomerase facilitates the unwinding activity of helicase.
Topoisomerase: The Unsung Hero of DNA Replication
As DNA replication unfolds, the double helix faces inevitable challenges. The tightly wound, intertwined strands create tension and supercoiling, hindering the unwinding process essential for replication. Enter topoisomerase, the unsung hero that resolves these structural dilemmas.
Topoisomerase is an enzyme that plays a pivotal role in relieving the tension and supercoiling that arise during DNA replication. It accomplishes this by breaking and rejoining the phosphodiester bonds that connect nucleotides in the DNA backbone. By doing so, topoisomerase creates temporary single-strand breaks, allowing the DNA helix to unwind and relax.
This unwinding process, facilitated by topoisomerase, is crucial for the smooth functioning of helicase, another key enzyme involved in DNA replication. Helicase is responsible for unwinding the DNA double helix, creating the replication fork where new DNA strands are synthesized. Without topoisomerase to relieve tension, helicase would encounter significant resistance in unwinding the DNA, potentially stalling the replication process.
Topoisomerase also assists in the removal of positive supercoils, which are tightly coiled regions of DNA that can impede replication. By breaking and rejoining DNA strands, topoisomerase allows these supercoils to relax, creating a more favorable environment for DNA replication to proceed.
In essence, topoisomerase is the silent guardian of DNA replication, ensuring that the intricate process of unwinding and copying the genetic material occurs seamlessly. Without its intervention, DNA replication would face insurmountable obstacles, rendering cell division and life itself impossible.
DNA Ligase: Joining the Fragments:
- Discuss the function of DNA ligase in joining the Okazaki fragments into a continuous strand.
- Explain how DNA ligase completes the DNA replication process.
DNA Ligase: The Master Seamster of DNA Replication
In the intricate world of DNA replication, where the blueprint of life is meticulously copied, there’s a crucial enzyme that acts like a master seamster—it’s called DNA ligase. Its mission is to stitch together the fragments of DNA, transforming them into a continuous and seamless blueprint.
After the DNA double helix unwinds during replication, the leading strand is synthesized continuously. However, the lagging strand, which runs in the opposite direction, faces a unique challenge. It’s synthesized in short segments called Okazaki fragments. These fragments overlap and need to be joined together precisely to create an unbroken strand.
That’s where DNA ligase steps in. This enzyme, like a tiny molecular thread, weaves its magic by catalyzing the covalent bonding of adjacent Okazaki fragments. It searches along the lagging strand, detecting gaps between the fragments. When it finds a gap, DNA ligase gracefully aligns the fragments and forms a phosphodiester bond, knitting them together.
With each stitch, DNA ligase tightens the grip on the DNA strand, ensuring its integrity and continuity. And so, the once-fragmented lagging strand is transformed into a seamless whole, ready to carry the genetic code to new cells.
The seamless joining of Okazaki fragments by DNA ligase marks the final step in DNA replication. It completes the creation of two identical daughter DNA molecules, each containing the same genetic information as the original DNA molecule. This process, repeated countless times throughout our cells, ensures the faithful transmission of genetic information from one generation to the next, safeguarding the continuity of life itself.