Dna Replication: The Crucial Foundation For Genetic Inheritance
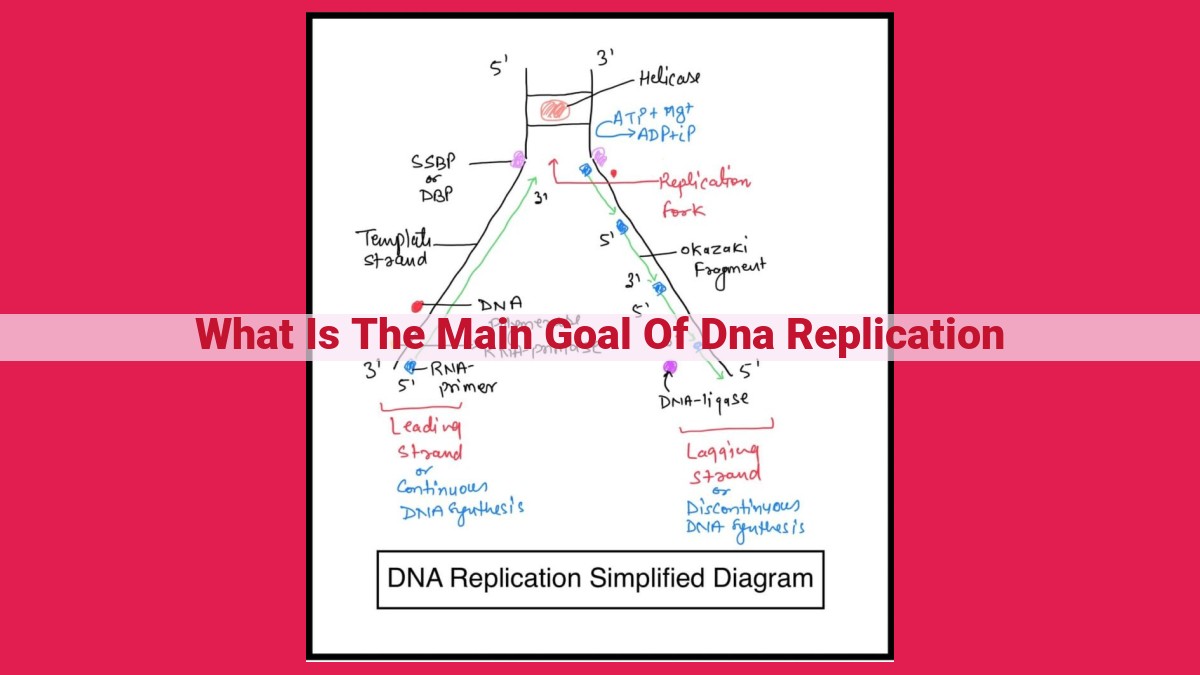
DNA replication, a fundamental process in cell division, ensures the accurate duplication of genetic information. By creating identical copies of DNA, replication enables the transmission of genetic material to daughter cells, maintaining genetic continuity and ensuring the proper functioning of living organisms.
DNA Replication: The Life-Giving Mechanism
DNA, the blueprint of life, holds the secrets of our genetic heritage and guides the symphony of life within every cell. DNA replication, the intricate process by which DNA makes an identical copy of itself, lies at the heart of cell division and the inheritance of life’s traits.
Semiconservative Replication: Precision Duplication
DNA replication is a masterful feat of precision, creating an exact replica of the original DNA molecule. This meticulous process, known as semiconservative replication, ensures that each daughter cell receives an identical copy of the genetic blueprint.
Ensuring Accuracy: Proofreading and Telomere Shortening
The accuracy of DNA replication is paramount for the integrity of genetic information. Proofreading enzymes, nature’s meticulous editors, meticulously check for and correct errors in the newly synthesized DNA strands. However, with each round of replication, telomeres, the protective caps at the ends of chromosomes, shorten. Over time, this shortening can contribute to cellular aging and the onset of age-related diseases.
Orchestrating the Replication Process
DNA replication is a coordinated symphony of events, involving a complex orchestra of enzymes and proteins. At the replication fork, the point where DNA unwinds, the leading strand is synthesized continuously, while the lagging strand is synthesized in short fragments, later joined together.
Implications for Human Health and Research
Mistakes in DNA replication can have profound consequences for human health, leading to genetic disorders and diseases such as cancer. Ongoing research in the field of DNA replication aims to unravel the mysteries of these errors, understand their implications for human health, and develop potential therapies. By delving into the intricate world of DNA replication, we unlock the secrets of life itself and pave the way for groundbreaking discoveries in medicine and beyond.
Semiconservative Replication: The Key to Accurate Genetic Inheritance
Picture this: you’re standing in a library, surrounded by countless books. To pass on your favorite stories to the next generation, you set out to make perfect copies of each book. This daunting task is not unlike what our cells encounter during DNA replication, the process that ensures every new cell inherits an exact replica of our genetic code.
DNA, the molecule that holds our genetic information, is a double helix composed of two strands complementary to each other. In semiconservative replication, each original strand serves as a template, guiding the formation of a new complementary strand.
During replication, the double helix separates into two single strands. Enzymes then move along these templates, adding nucleotides (the building blocks of DNA) one by one. Each newly synthesized strand pairs with its complementary template strand, resulting in two identical copies of the original DNA molecule.
This remarkable process ensures that genetic information is passed accurately every time a cell divides. The precision of DNA replication is vital for life. Errors can lead to mutations, which in turn may cause diseases like cancer.
The importance of accuracy is further highlighted by telomeres, specialized structures that protect the ends of chromosomes. Telomeres shorten with each cell division. When they become too short, replication becomes compromised, potentially leading to cell death or cancer.
Ensuring Accuracy in DNA Replication: Proofreading and Telomere Shortening
In the intricate dance of life, DNA replication stands as a keystone process, ensuring the precise duplication of genetic material as cells divide and proliferate. Accuracy is paramount in this delicate operation, as any deviations can have profound implications for cellular health and organismal well-being.
Proofreading Enzymes: Guardians of Genetic Fidelity
As the replication machinery meticulously copies the DNA template, proofreading enzymes diligently scan the newly synthesized strands, meticulously identifying and correcting any errors that may have crept in during the process. These molecular sentinels, such as polymerase delta and mismatch repair proteins, act as vigilant guardians of genetic integrity, ensuring that the DNA blueprints remain pristine.
Telomere Shortening: A Balancing Act of Life and Aging
At the ends of each chromosome lie specialized DNA sequences called telomeres. These protective caps serve as buffers during replication, preventing the erosion of essential genetic information. However, with each cell division, telomeres gradually shorten, a natural consequence of the replication process. Over time, this shortening can reach a critical threshold, triggering cellular senescence or apoptosis—a programmed cell death that prevents damaged cells from proliferating.
Implications for Health and Research
DNA replication errors and telomere shortening are not merely academic curiosities; they have tangible consequences for human health. Errors in replication can lead to mutations, which may contribute to the development of cancer and other genetic disorders. Telomere shortening has been linked to aging-related diseases such as cardiovascular disease and Alzheimer’s disease.
Ongoing research into the mechanisms of proofreading and telomere shortening holds great promise for advancing our understanding of aging, disease, and cancer. Scientists are exploring novel strategies to enhance proofreading efficiency and extend telomere length, offering potential avenues for therapeutic interventions in the future.
Delving into the Replication Process: A Coordinated Effort
At the heart of cell division and genetic inheritance lies a meticulous process known as DNA replication. During this intricate dance, the double helix of DNA unfurls, revealing its hidden secrets, in an effort to create identical copies of itself. This vital process ensures that the delicate symphony of genetic information is passed accurately from generation to generation.
The replication process commences with the unwinding of the DNA helix, revealing two distinct strands, much like a zipper being unzipped. Along each exposed strand, a replication fork emerges, acting as a gateway for the replication machinery to initiate its task. The replication fork comprises a complex ensemble of enzymes, each playing a crucial role in orchestrating this intricate procedure.
As the replication fork progresses, two new strands of DNA are synthesized, each meticulously following the sequence of the original strand, akin to a meticulous scribe copying a precious manuscript. The newly formed strands remain entwined with their respective templates, creating two leading strands and two lagging strands.
The synthesis of leading strands is a relatively straightforward process, as they can be extended continuously in the same direction as the replication fork. Lagging strands, on the other hand, face a unique challenge. Due to the antiparallel nature of DNA, they must be synthesized in short fragments, known as Okazaki fragments, which are later joined together by a specialized enzyme called DNA ligase.
The precision and accuracy of DNA replication is paramount, as even a single error could disrupt the genetic code and potentially lead to devastating consequences. To ensure the utmost fidelity, DNA polymerases, the molecular maestros of replication, possess an in-built proofreading ability. These enzymes meticulously check each newly added nucleotide, ensuring its correct pairing with the template strand.
Furthermore, at the ends of chromosomes lie protective caps called telomeres, which prevent the loss of essential genetic information during repeated cell divisions. However, with each cell division, telomeres shorten, eventually triggering cellular senescence or apoptosis, a form of programmed cell death. This natural process contributes to aging and is implicated in age-related diseases.
Understanding the intricacies of DNA replication is not merely an academic pursuit but holds profound implications for human health and medical research. Errors in DNA replication can result in genetic diseases, while alterations in telomere length have been linked to aging-related conditions. Ongoing research in these areas aims to uncover novel therapeutic strategies and therapies that could potentially mitigate these effects.
As we continue to unravel the complexities of DNA replication, we gain a deeper appreciation for the remarkable mechanisms that sustain life and ensure the continuity of genetic information across generations.
Implications for Human Health and Research
DNA Replication Errors and the Scourge of Disease
The precision of DNA replication is paramount for maintaining the integrity of our genetic material. When errors occur during this intricate process, they can have dire consequences for human health. DNA replication errors can lead to mutations, which are changes in the DNA sequence that can alter gene function. Mutations can contribute to the development of diseases such as cancer, genetic disorders, and premature aging.
Telomere Shortening: The Ticking Clock of Aging
Telomeres are specialized DNA structures that cap the ends of chromosomes, protecting them from degradation. With each cell division, telomeres shorten. This gradual loss of telomeres ultimately limits the number of times a cell can divide, a phenomenon known as the Hayflick limit. As telomeres become critically short, cells enter a state of senescence, losing their ability to divide and contribute to tissue regeneration. Telomere shortening is a key factor in the aging process and is linked to age-related diseases such as cardiovascular disease, neurodegenerative disorders, and cancer.
Ongoing Research: Paving the Way for New Therapies
The profound implications of DNA replication errors and telomere shortening for human health have spurred extensive research efforts. Scientists are delving into the complex mechanisms that regulate these processes, seeking to identify new targets for therapeutic interventions. Ongoing research aims to develop strategies for preventing or correcting DNA replication errors, as well as therapies to mitigate the effects of telomere shortening.
Advancements in Cancer Treatment: Targeting DNA Replication
One promising area of research focuses on targeting DNA replication errors in cancer cells. By inhibiting DNA polymerases, the enzymes responsible for DNA synthesis, scientists hope to exploit the inherent genomic instability of cancer cells. Such approaches have shown promise in preclinical and clinical studies, paving the way for potential new cancer treatments.
Telomere-Based Therapies: Extending Lifespans
Another exciting frontier in research revolves around telomere-based therapies. By manipulating telomerase, the enzyme that synthesizes telomeres, scientists aim to extend telomere length and promote cell longevity. This approach has garnered interest for potential applications in anti-aging interventions and the treatment of age-related diseases.
The study of DNA replication and telomere shortening holds immense promise for improving human health. As researchers continue to unlock the secrets of these fundamental processes, we move closer to developing novel therapies that combat disease and promote longevity. The implications of this research extend far beyond the laboratory, shaping the future of medicine and our understanding of the human condition.