Understanding Dna Replication: Key Concepts And Mechanisms
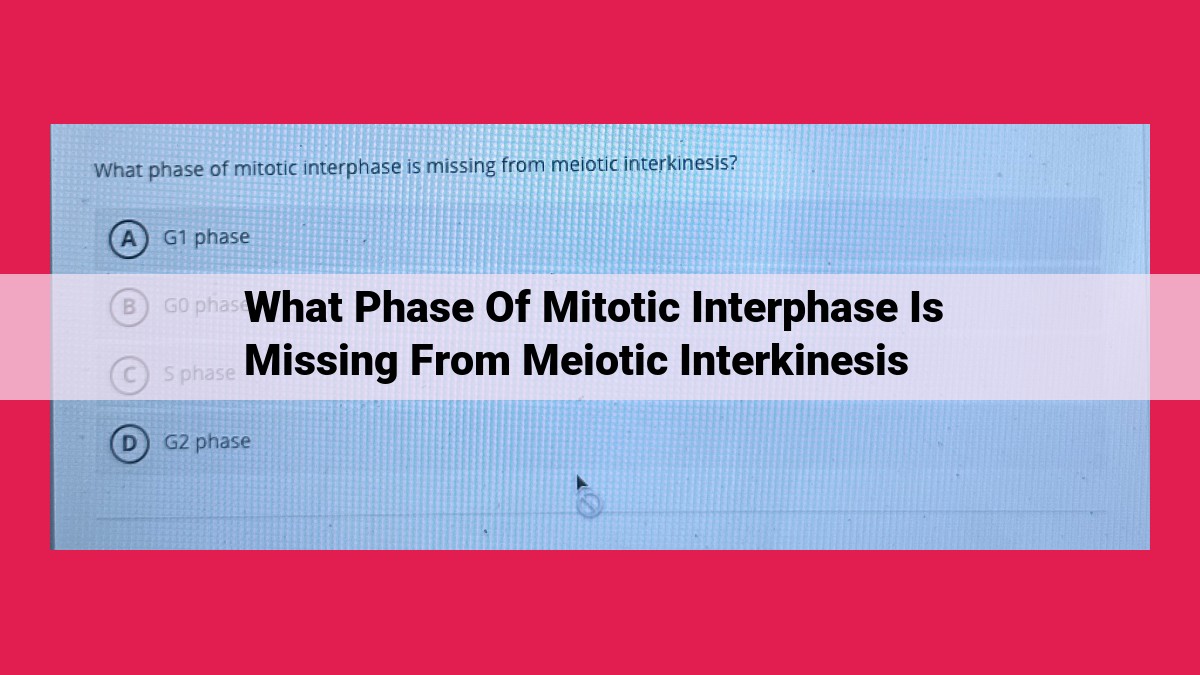
- Introduction to DNA Replication
- Key Enzymes in DNA Replication
- The Replication Fork
- Leading and Lagging Strands
- Okazaki Fragments and DNA Ligase
- Conclusion
The paragraph you provided does not contain any information about mitotic interphase or meiotic interkinesis. Therefore, I cannot create a description of “what phase of mitotic interphase is missing from meiotic interkinesis” using the given text.
Unveiling the Secrets of DNA Replication: The Blueprint for Life
In the intricate dance of life, DNA replication is the choreographer, ensuring the precise transmission of genetic information from generation to generation. It’s a biological marvel that underpins every aspect of our existence, from the tiniest of cells to the grandest of organisms.
The Importance of DNA Replication
DNA, or deoxyribonucleic acid, is the building block of life, carrying the genetic blueprint that defines our physical traits and biological functions. Replication is the process by which this genetic code is faithfully copied, enabling cells to divide, grow, repair, and pass on their genetic legacy. It’s crucial for the continuity of all living organisms.
Key Enzymes in DNA Replication: The Unsung Heroes of Cellular Life
In the grand symphony of life, DNA replication stands as a maestro, orchestrating the precise duplication of genetic information essential for cell division, growth, repair, and heredity. Behind the scenes of this intricate process, a symphony of enzymes plays a critical role, each with a unique talent that ensures the faithful transmission of genetic material.
The Star of the Show: DNA Polymerase
DNA polymerase takes center stage as the molecular machine responsible for synthesizing new DNA strands. As it diligently reads the existing DNA template, it flawlessly assembles complementary nucleotides (think of these as Lego blocks), meticulously adding them to the growing chain.
But DNA polymerase is not merely a blind builder. It possesses an astute skill known as “proofreading.” After each nucleotide is added, it meticulously checks its work, ensuring that no errors have crept into the sequence. This quality control ensures that the genetic code is copied with unrivaled precision.
Unwinding the Double Helix: The Role of Helicase
The DNA double helix, a tightly intertwined spiral staircase, presents a formidable obstacle to replication. Enter helicase, an enzyme that acts like a molecular crowbar, prying apart the strands and uncoiling the helix. This unwinding creates a “replication bubble,” providing the necessary space for DNA polymerases to work their magic.
Relieving the Twist: Topoisomerase Steps Up
As the DNA helix unwinds, it creates a buildup of torsional stress, akin to a tightly wound rubber band. Topoisomerase comes to the rescue, acting as a molecular barber, strategically cutting and reattaching the DNA strands to relieve the tension and allow replication to proceed smoothly.
In Summary
These key enzymes—DNA polymerase, helicase, and topoisomerase—play indispensable roles in DNA replication, the cornerstone of cellular life. Their intricate interplay ensures the precise duplication of genetic information, safeguarding the integrity of our genetic code and allowing life to flourish.
The Replication Fork: Where DNA Replication Takes Center Stage
In the bustling metropolis of the cell, DNA replication is a mesmerizing dance that ensures the integrity of life. And at the heart of this dance lies a crucial location known as the replication fork. Picture a Y-shaped juncture, where the double helix of DNA gracefully unravels, making way for a choreographed symphony of replication.
At this molecular crossroads, two strands of DNA, each bearing a unique code of life, gracefully separate, creating two replication bubbles. Here, nature’s master architects, enzymes, orchestrate a delicate ballet of synthesis.
One strand, the leading strand, embarks on a continuous journey of replication, synthesizing new DNA in the 5′ to 3′ direction. Like a tireless marathon runner, it marches steadily, adding nucleotide building blocks one by one.
The other strand, the lagging strand, faces a more complex task. It must replicate in the opposite direction, 5′ to 3′, but due to the antiparallel nature of DNA, it cannot proceed continuously. Instead, it forms a series of short, single-stranded fragments called Okazaki fragments.
Like puzzle pieces, these Okazaki fragments are temporarily held in place by special proteins until DNA ligase, the molecular glue of the cell, arrives to stitch them together into a cohesive, continuous strand. This intricate dance at the replication fork ensures that each new cell receives an exact copy of the original DNA, preserving the precious genetic blueprint that guides all life.
Leading and Lagging Strands: The Unfolding Tale of DNA Replication
As we delve into the intricate world of DNA replication, a captivating story unfolds, one that reveals the remarkable precision and coordinated dance of cellular machinery. At the heart of this process lie two ingenious strands, known as the leading and lagging strands, each playing a unique role in the meticulous copying of our genetic blueprint.
The Leading Strand: A Seamless Unfolding
Picture the leading strand as a tireless explorer, embarking on a continuous journey along the DNA helix. With unwavering determination, it unwinds the double helix using the guiding hand of helicase, the enzyme that acts as a molecular zipper. As it traverses the exposed template, DNA polymerase diligently weaves a complementary strand, adding nucleotides one by one in an uninterrupted flow, much like a tireless seamstress stitching an intricate tapestry.
The Lagging Strand: A Rhythmic Dance
The lagging strand, in contrast, faces a more intricate challenge. As the DNA helix unwinds ahead of it, the lagging strand is forced to chase after the receding template. Unable to embark on a continuous synthesis, it proceeds in a series of rhythmic leaps and bounds, creating a sequence of short, single-stranded fragments called Okazaki fragments.
Each Okazaki fragment, once synthesized by DNA polymerase, is handed off to the watchful care of DNA ligase, the molecular glue that seals the gaps between them, creating a continuous, cohesive strand. It’s like a team of nimble acrobats, each performing their part with precision, ensuring the seamless completion of the lagging strand.
The Interplay of the Leading and Lagging Strands: A Symphony of Replication
Together, the leading and lagging strands orchestrate a harmonious symphony of replication. The continuous synthesis of the leading strand paves the way for the more complex, discontinuous nature of the lagging strand’s assembly. Their combined efforts ensure the accurate duplication of our genetic material, a critical step for the growth, repair, and propagation of all living organisms.
Okazaki Fragments and DNA Ligase: Ensuring the Continuity of the Lagging Strand
As the replication fork progresses, the lagging strand faces a unique challenge. Its synthesis occurs in the opposite direction of the fork movement, resulting in discontinuous fragments known as Okazaki fragments. These fragments are short, single-stranded DNA segments ranging from 100 to 200 nucleotides in length.
The intricate process of DNA replication depends on the synchronized action of enzymes to ensure the accuracy and continuity of the newly synthesized DNA strands. Among these enzymes, DNA ligase plays a crucial role in bridging the gaps between Okazaki fragments, stitching them together to form a cohesive, continuous lagging strand.
DNA ligase, a ligase enzyme, possesses the remarkable ability to form phosphodiester bonds between the 3′ hydroxyl group of one fragment and the 5′ phosphate group of the next. This process occurs repeatedly, connecting the Okazaki fragments into a continuous strand on the lagging side.
The seamless joining of Okazaki fragments is essential for maintaining the integrity of the newly synthesized DNA molecule. Without DNA ligase, these fragments would remain isolated, impairing the essential cellular processes that rely on continuous DNA strands.