Unveiling The Molecular Secrets Of Life: A Comprehensive Guide To Dna’s Role In Replication, Transcription, And Translation
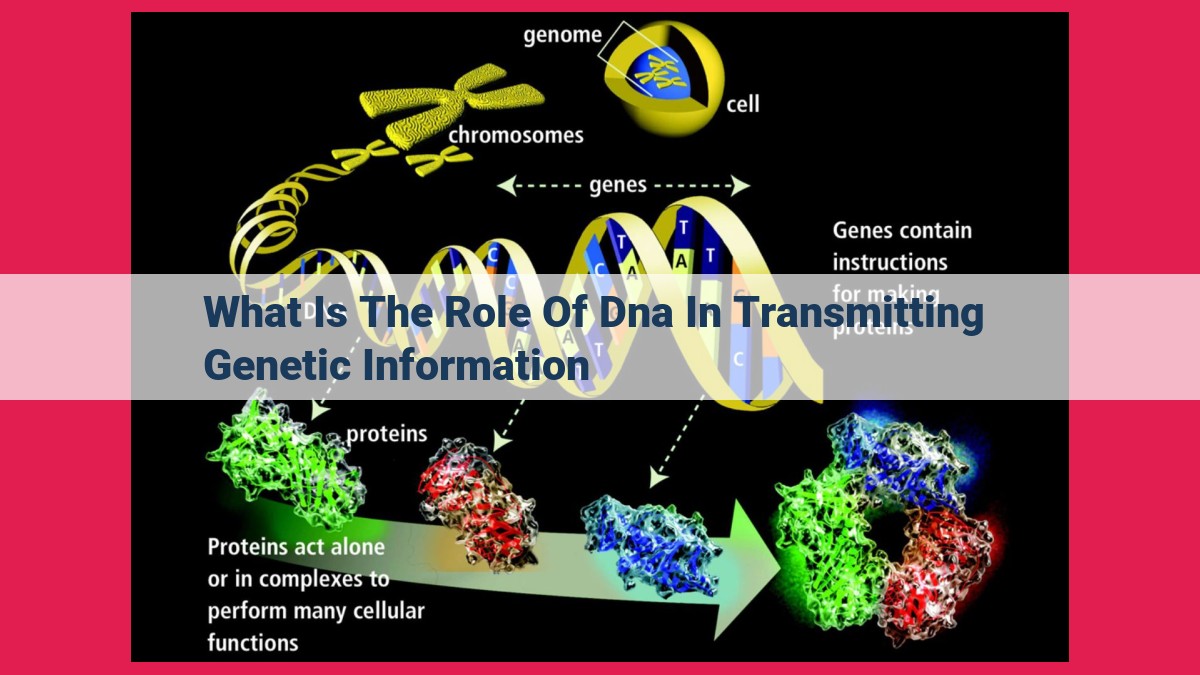
DNA, the molecule of life, carries genetic information in its structure, consisting of nucleotides arranged in a double helix. Through replication, DNA copies itself to pass on genetic material to new cells. In transcription, DNA serves as a template for RNA synthesis, which carries the genetic code to ribosomes. Translation utilizes RNA and ribosomes to synthesize proteins, which are the building blocks of life. These processes ensure the precise transmission of genetic information, enabling cell division and inheritance of traits.
DNA: The Blueprint of Life
In the grand tapestry of life, DNA stands as the master molecule, the blueprint from which all living organisms are woven. This intricate molecule is the repository of our genetic inheritance, the architect of our physical traits, and the custodian of our evolutionary destiny.
At its core, DNA is a double helix composed of nucleotides, the building blocks of genetic information. These nucleotides, in turn, are composed of a sugar molecule, a phosphate group, and one of four nitrogenous bases: adenine (A), thymine (T), cytosine (C), and guanine (G). The sequence of these bases along the DNA molecule forms the genetic code, the language of life that determines our unique characteristics.
The double helix structure of DNA not only protects the genetic code but also provides the framework for the molecule’s replication. During cell division, the DNA molecule unwinds and separates, each strand serving as a template for the synthesis of a new complementary strand. This semiconservative replication ensures that each daughter cell inherits an identical copy of the genetic material, preserving the continuity of life.
Unveiling DNA’s Structure
- Describe the composition of DNA, including nucleotides, base pairs, and the double helix.
- Explain the significance of DNA’s structure in maintaining genetic information.
Unveiling DNA’s Structure: Unraveling the Blueprint of Life
The Building Blocks of Life: Nucleotides
At the core of our very existence lies deoxyribonucleic acid (DNA), the enigmatic molecule that carries the blueprints for life. DNA comprises microscopic building blocks known as nucleotides, each consisting of a sugar molecule, a phosphate group, and a nitrogenous base – the essential components that dictate our genetic heritage. There are four types of nitrogenous bases: adenine (A), thymine (T), cytosine (C), and guanine (G).
The Double Helix: A Masterpiece of Molecular Architecture
Imagine a spiraling staircase, its intricate steps meticulously entwined – this is the iconic double helix structure of DNA. Each step is a base pair, a perfect union between two complementary bases: A always pairs with T, and C with G. This exquisite arrangement, discovered by scientists James Watson and Francis Crick, forms the backbone of the DNA molecule.
The Significance of DNA’s Structure
The double helix structure is not merely an aesthetic wonder but a testament to DNA’s remarkable ability to store and transmit genetic information. The order of base pairs along the DNA strand forms unique sequences, akin to the letters of an intricate code. These sequences encode the instructions for every aspect of an organism, from its physical traits to its susceptibility to disease.
By understanding the structure of DNA, scientists have unlocked the secrets of life, revolutionizing our understanding of genetics, medicine, and countless other fields.
The Art of Replication: Copying DNA
- Describe the semiconservative nature of DNA replication.
- Introduce DNA polymerase as the enzyme that synthesizes new DNA strands.
- Explain the concept of replication forks.
The Art of Replication: Copying DNA
Imagine DNA as a precious recipe book, holding the exquisite blueprint of life. Now, picture yourself as a meticulous chef tasked with duplicating this culinary masterpiece. That’s precisely what cells do during DNA replication, a process that ensures every new cell receives an exact copy of the genetic code.
Replication follows a semiconservative approach, meaning each new DNA molecule inherits one strand from the parent molecule and synthesizes a complementary new strand. Enter DNA polymerase, the molecular maestro that orchestrates this replication symphony.
As DNA polymerase glides along the original template strand, it reads the sequence of nucleotides (the building blocks of DNA) like musical notes. Using these cues, it meticulously adds complementary nucleotides to create a new, daughter strand. This process unfolds simultaneously on both strands of the DNA double helix, giving rise to two identical copies.
Imagine a replication fork, a molecular Y-shaped region, where the unwound DNA helix serves as a stage for DNA polymerase’s meticulous performance. The fork gradually moves along the DNA molecule, unzipping the helix and allowing polymerase to work its magic.
Transcription: DNA to RNA
- Introduce RNA polymerase and its role in initiating RNA synthesis.
- Discuss promoters as DNA regions that signal the start of transcription.
- Explain the process of RNA splicing to remove non-coding regions.
Transcription: Unraveling the DNA Blueprint into RNA
We’ve delved into the enigmatic world of DNA, the blueprint that holds the secrets of life. Now, let’s embark on the next chapter of this molecular saga: transcription, the process that translates DNA’s genetic instructions into RNA.
Imagine RNA polymerase, the maestro of transcription, binding to a specific region of DNA called the promoter. This musical cue signals the start of a symphony of molecular events. As RNA polymerase glides along the DNA, it unwinds the double helix, exposing the nucleotide sequence.
With this exposed DNA as its template, RNA polymerase begins its synthesis of RNA. Nucleotides, the building blocks of RNA, are carefully matched to their complementary bases on DNA. Like a meticulous composer writing a score, RNA polymerase assembles a new RNA strand, mirroring the DNA sequence.
As the RNA strand is synthesized, it’s a bit of a rough draft. It contains both coding and non-coding regions, like paragraphs and footnotes in a book. To create a more polished version, the RNA strand undergoes a process called splicing. Here, enzymes remove the non-coding regions, leaving behind only the essential genetic information.
The resulting RNA strand is now known as messenger RNA (mRNA). Like a messenger carrying an important dispatch, mRNA transports the genetic instructions from the nucleus, where DNA resides, to the cytoplasm, where protein synthesis occurs. It’s like the blueprint being sent to the construction site.
Transcription is a vital stage in the flow of genetic information. It’s through this process that the instructions encoded in DNA are transcribed into RNA, the intermediary molecule that carries the genetic code to the protein-making machinery of cells. Without transcription, no proteins could be made, and our bodies would cease to function.
Translation: RNA to Protein – The Synthesis Symphony
In the bustling metropolis of the cell, where the secrets of life unfold, we embark on a journey to unravel the intricate process of translation – the miraculous conversion of RNA’s blueprint into the building blocks of life, proteins.
Ribosomes: The Protein Assembly Line
Picture ribosomes, the molecular machines responsible for protein synthesis. These intricate structures reside within the cell’s cytoplasm, patiently awaiting the arrival of their molecular cargo. When the messenger RNA (mRNA), carrying the precise instructions from DNA, reaches the ribosome, the symphony of protein synthesis begins.
Transfer RNA: The Amino Acid Messengers
Like tiny shuttles, transfer RNAs (tRNAs) scurry about the cell, each carrying a specific amino acid – the fundamental units of proteins. Guided by anticodons, their complementary sequences to mRNA codons, tRNAs dock at the ribosome, delivering their precious cargo.
Amino Acids: The Protein Building Blocks
As tRNAs align with mRNA codons, amino acids are linked together, forming a polypeptide chain – the nascent protein. This chain grows one amino acid at a time, dictated by the mRNA sequence. Each amino acid contributes its unique properties, shaping the protein’s structure and function.
The Dance of Proteins
Once complete, the polypeptide chain detaches from the ribosome and embarks on its journey to become a functional protein. It folds and twists into a specific shape, interacting with other molecules to perform its vital tasks. Proteins are the workhorses of the cell, essential for everything from metabolism and movement to communication and defense.
The Importance of Translation
Translation is the bridge between genetic information and the proteins that power life. It enables cells to synthesize a vast array of proteins, each tailored to specific functions. Without translation, the cell would be unable to maintain its intricate machinery, adapt to changing conditions, or carry out its myriad tasks.
Translation, the final step in the central dogma of molecular biology, is an awe-inspiring process that brings the blueprint of life to fruition. It is a testament to the incredible complexity and beauty of the molecular world within us.
The Genetic Code: Unraveling the Language of Life
Imagine DNA as the blueprint for all living organisms, holding the instructions for every trait and characteristic. Within this microscopic masterpiece lies a hidden language, the genetic code, which translates the blueprints into the proteins that build and sustain life.
At the heart of this code are codons, sequences of three nucleotides (the building blocks of DNA) that specify which amino acid (the building blocks of proteins) will be added next. Each codon represents a specific amino acid, forming the alphabet of life’s genetic language.
To decipher this language, cells rely on a molecule called transfer RNA (tRNA). Each tRNA molecule carries an anticodon, which is complementary to a specific codon on the messenger RNA (_mRNA)—an intermediary molecule that carries the DNA instructions to ribosomes, the protein-making machines of cells.
When a tRNA with a matching anticodon binds to a codon on the mRNA, it delivers the corresponding amino acid to the ribosome. As the ribosome reads the codons in sequence, it assembles the amino acids into a polypeptide chain, ultimately forming the protein encoded by the DNA.
Variations in the genetic code are responsible for the incredible diversity of life on Earth. Changes in the sequence of codons can alter the amino acid sequence of proteins, leading to different traits and characteristics. These variations can arise from mutations, random changes in the DNA that can have profound effects on the resulting proteins.
By understanding the genetic code, scientists have gained unprecedented insight into the very essence of life. Its decoding has revolutionized our ability to diagnose and treat genetic diseases, develop new therapies, and unravel the mysteries of evolution. The genetic code is a testament to the intricate complexity and awe-inspiring beauty of the natural world.
Unplanned Tweaks in the Genetic Blueprint: Mutations
What are Mutations?
Imagine DNA, the blueprint of life, as a sacred text etched in nucleotide letters. Mutations are like unforeseen typos in this text, altering the genetic code in ways that can have profound implications. These spontaneous changes in DNA can be as subtle as a single nucleotide swap or as dramatic as insertions or deletions that shift the entire genetic landscape.
Types of Mutations
-
Point Mutations: Think of a single typo in a novel – a base pair substitution that swaps one nucleotide for another. These minor tweaks can alter the amino acid sequence of a protein, potentially affecting its function.
-
Frameshift Mutations: Imagine deleting or inserting entire words in a sentence. Frameshift mutations do the same to DNA, shifting the reading frame and potentially scrambling the entire protein sequence. These drastic alterations can have severe consequences.
-
Spontaneous Mutations: The vast majority of mutations occur spontaneously, like cosmic rays striking our DNA. These random changes can arise from a variety of factors, including environmental toxins and the inevitable errors of DNA replication.
The Impact of Mutations
Mutations can have a spectrum of effects, ranging from beneficial to harmful. Some mutations may even be neutral, with no noticeable impact on the organism.
-
Neutral Mutations: Like harmless typos that don’t alter the meaning of a sentence, these mutations have no discernible effect.
-
Beneficial Mutations: Occasionally, mutations can be like serendipitous discoveries – they introduce positive changes in a gene, enhancing an organism’s survival or reproductive success.
-
Harmful Mutations: Unfortunately, many mutations are detrimental, causing diseases or disorders. These alterations can disrupt protein function, alter gene regulation, or even lead to genetic diseases like cystic fibrosis or sickle cell anemia.
The Importance of Mutations
Despite their potential risks, mutations are crucial drivers of evolution. They provide the raw genetic material upon which natural selection can act. Mutations create genetic diversity, allowing populations to adapt to changing environments and giving rise to new species.
Mutations, like unplanned tweaks in the genetic blueprint, are both a source of danger and opportunity. They can introduce disease, but they can also spark the spark of evolution. Understanding mutations is essential for unraveling the mysteries of life, from the origins of our species to the development of genetic therapies.