Optimized Title: Dna: Unveiling The Building Blocks Of The Genetic Code
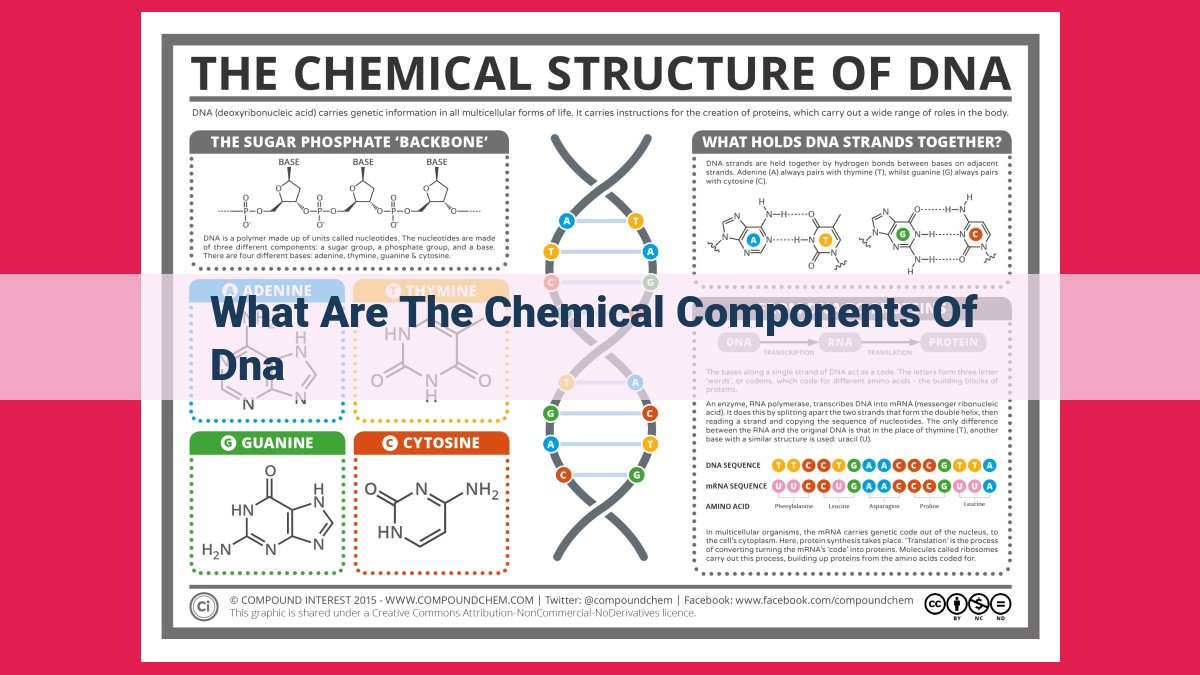
DNA, the genetic blueprint, is made up of nucleotides—the building blocks composed of phosphate, deoxyribose sugar, and nitrogenous bases. The phosphate backbone provides a negative charge, while the sugar forms the backbone’s structural support. The four nitrogenous bases—adenine, thymine, cytosine, and guanine—form specific base pairs, encoding the genetic code and contributing to the double helix structure. This iconic shape facilitates DNA replication, repair, and protection of genetic information, showcasing the crucial role of these chemical components in the foundation of life.
The Genetic Blueprint: DNA’s Essential Chemical Components
Meet the master molecule of life, deoxyribonucleic acid (DNA), the blueprint that encodes the genetic instructions for every living organism on Earth. From the simplest bacteria to towering trees and the complexity of human beings, DNA holds the secrets to our existence. It’s a complex molecule, but understanding its chemical components is crucial to unraveling the mysteries of life.
Nucleotides: The Building Blocks of DNA
Imagine DNA as a giant jigsaw puzzle, and nucleotides are the individual pieces that make up the whole. Each nucleotide is made up of three parts: a phosphate, a sugar, and a nitrogenous base. The sequence of these bases along the DNA molecule is what determines the genetic code.
The Double Helix: DNA’s Iconic Structure
Now, picture two strands of these nucleotides intertwined like a twisted ladder. This is called the double helix, the iconic shape of DNA that grants it remarkable stability and flexibility. The rungs of this ladder are formed by base pairs, with adenine always matching with thymine, and cytosine with guanine. This specific pairing ensures that the genetic code is accurately preserved and replicated.
The Role of Phosphate and Sugar
The phosphate group, with its negative charge, forms the backbone of the DNA molecule, providing structural support and stability. The sugar molecule, called deoxyribose, links the nucleotides together, creating the backbone’s structural scaffold.
Nitrogenous Bases: The Genetic Code
The nitrogenous bases are the true stars of DNA’s genetic code. Adenine (A), thymine (T), cytosine (C), and guanine (G) form the four letters of this genetic alphabet. Their arrangement along the DNA molecule determines our inherited traits and characteristics.
The chemical components of DNA – nucleotides, phosphate, sugar, and nitrogenous bases – work in harmony to create a molecule that is both information-rich and remarkably resilient. It is the foundation of life, carrying the instructions for our development, growth, and reproduction. Understanding the chemistry of DNA is not just a scientific pursuit; it’s a journey into the very essence of our existence.
Nucleotides: The Fundamental Units of DNA, the Blueprint of Life
In the intricate tapestry of life, DNA stands as the genetic blueprint, orchestrating the very fabric of our existence. Its chemical structure, a complex symphony of components, forms the foundation for this vital molecule. Among these essential elements are nucleotides, the fundamental units of DNA, the building blocks upon which the genetic code is constructed.
Each nucleotide is a captivating fusion of three distinct components: a phosphate group, a sugar molecule, and a nitrogenous base. The phosphate group, with its negatively charged nature, plays a pivotal role in shaping the DNA molecule’s structure. It forms the backbone of the DNA double helix, linking nucleotides together in a chain-like formation.
The sugar molecule, a five-carbon structure known as deoxyribose, provides the necessary framework for the nucleotide. It forms the backbone of the DNA molecule and provides flexibility, allowing the DNA to bend and flex without compromising its integrity.
Finally, the nitrogenous base, the most captivating component, holds the secret to DNA’s genetic code. There are four distinct nitrogenous bases in DNA: adenine (A), thymine (T), cytosine (C), and guanine (G). These bases pair up in specific combinations – A with T, and C with G – to form the “rungs” of the DNA ladder. This base pairing, the cornerstone of DNA’s genetic code, determines the sequence of amino acids in proteins, the building blocks of all living organisms.
Deoxyribonucleic Acid (DNA): A Double Helix
- Describe the double helix structure and how it is formed by linking nucleotides.
- Explain the significance of this structure for DNA stability and function.
Deoxyribonucleic Acid (DNA): The Double Helix Masterpiece
In the realm of life’s blueprint, Deoxyribonucleic Acid (DNA) stands as the iconic molecule that holds the secrets to our genetic code. Its intricate structure, known as the double helix, is a marvel of nature, providing stability and functionality to this vital molecule.
Imagine a spiral staircase gracefully winding upward, each step representing a nucleotide, the essential building block of DNA. Nucleotides interlock, forming the two intertwined strands of the double helix through hydrogen bonds between specific nitrogenous bases. The phosphate backbone, composed of negatively charged molecules, forms the outer edges of the helix, providing structural support.
The deoxyribose sugar, with its unique shape, constitutes the backbone’s framework, ensuring both stability and flexibility. Within the helix, four nitrogenous bases—adenine (A), thymine (T), cytosine (C), and guanine (G)—hold the key to the genetic code. Specific base pairs, A-T and C-G, complement each other, forming the rungs of the helical ladder.
This double helix conformation imparts remarkable stability to DNA. The complementary strands interact seamlessly, allowing for replication, repair, and protection of the genetic information encoded within. The hydrogen bonds between base pairs maintain the structural integrity of the double helix, safeguarding the delicate blueprint of life.
Thus, DNA’s double helix embodies the ingenious design of nature. Its chemical composition and intricate structure enable the storage, transmission, and expression of genetic information, ensuring the continuity of life across generations.
Phosphate: The Negatively Charged Backbone
The chemical structure of deoxyribonucleic acid (DNA) plays a pivotal role in its role as the blueprint of life. Among the essential components of DNA, phosphate stands out as the negatively charged backbone that contributes to its unique double helix structure and stability.
Phosphate is found in the backbone of DNA, along with deoxyribose sugar and nitrogenous bases. It is a negatively charged molecule, meaning it has an excess of electrons. This negative charge repels other negatively charged molecules, which helps to stabilize the DNA molecule.
The phosphate groups are linked together by phosphodiester bonds, forming a chain that runs along the length of the DNA molecule. This chain of phosphate groups provides the backbone for the double helix structure, which is formed by two strands of DNA twisted around each other.
The negative charge of the phosphate backbone also attracts positively charged ions*, such as sodium and magnesium, which **help to neutralize the negative charge and stabilize the DNA molecule. These ions are found in the surrounding environment of the DNA molecule and contribute to its overall stability.
In summary, the phosphate groups in DNA form the negatively charged backbone that contributes to the double helix structure and stability of the DNA molecule. This backbone provides the framework for the genetic information that is encoded in the sequence of nitrogenous bases along the DNA molecule.
Sugar (Deoxyribose): The Structural Backbone of DNA
The intricate masterpiece of DNA, the blueprint of life, is not merely a collection of random chemicals. Each component plays a crucial role in the molecule’s structure and function. Deoxyribose, a sugar molecule, forms the foundational backbone of DNA, providing it with stability and flexibility.
Deoxyribose, unlike its cousin ribose found in RNA, lacks an oxygen molecule at the 2′ carbon position. This subtle difference has profound implications for the DNA molecule. It renders the sugar molecule more rigid, allowing it to form a stronger and more stable backbone compared to RNA.
The deoxyribose backbone is a linear chain, with each sugar molecule linked to the next through phosphate groups. The phosphate groups carry a negative charge, imparting an overall negative charge to the DNA molecule. This negative charge helps neutralize the positive charges of associated proteins and ions, stabilizing the DNA structure and protecting it from enzymatic degradation.
Furthermore, the deoxyribose sugar provides flexibility to the DNA molecule. Its rigid structure allows for bending and twisting, crucial for processes such as DNA replication, repair, and the formation of nucleosomes, the structural units of chromosomes. Without this flexibility, DNA would be brittle and prone to breakage.
In essence, deoxyribose sugar forms the堅牢で柔軟な支柱 of DNA, providing the molecule with stability and flexibility. These properties are instrumental in protecting the genetic information encoded within DNA, allowing it to faithfully transmit and express the blueprint of life from one generation to the next.
Nitrogenous Bases: The Enigmatic Code of DNA
The enigmatic code of DNA, the blueprint of life, lies within its chemical components. Among these elements, the nitrogenous bases hold the key to its genetic language.
The Quartet of Life
The nitrogenous bases—adenine (A), thymine (T), cytosine (C), and guanine (G)—form the foundation of genetic information. These chemical messengers carry the instructions that determine the traits of all living organisms.
The Dance of Base Pairing
Within the double helix structure of DNA, nitrogenous bases engage in a complementary dance, forming specific pairs. Adenine always seeks out thymine, while cytosine exclusively binds with guanine. This pairing creates the rungs of the DNA ladder and maintains the structural integrity of the molecule.
The Language of Inheritance
The sequence of these base pairs along the DNA strands constitutes the genetic code. This code is passed down from generation to generation, providing the inheritance of traits and ensuring the continuity of species. Each sequence of three nitrogenous bases (a codon) codes for a specific amino acid, the building blocks of proteins.
The Replication Machine
During cell division, DNA’s strand separation triggers a remarkable process called replication. The nitrogenous bases act as templates, guiding the formation of complementary strands. This flawless duplication ensures the accurate transmission of genetic information to daughter cells.
The Protectors of the Code
The base pairing of nitrogenous bases also plays a crucial role in DNA’s stability. The strong bonds between complementary pairs protect the genetic code from external threats, such as radiation and chemical damage. This stability is essential for the preservation of life’s blueprint.
The Chemical Foundation of Life
In conclusion, the nitrogenous bases of DNA are the cornerstone of the genetic code. Their specific pairing, dance-like in nature, determines the inheritance of traits and ensures the continuity of life. As the chemical foundation of our very existence, these enigmatic messengers hold the secrets to our biological heritage.
Double Helix: The Iconic Shape of DNA
DNA’s remarkable double helix structure is not merely an artistic marvel; it holds profound functional significance. This elegant architecture, resembling a twisted ladder, is the cornerstone of DNA’s ability to safeguard and transmit genetic information with unmatched precision.
The double helix is formed by the intricate interplay of its chemical constituents. Nitrogenous bases, the “language” of heredity, pair up in a highly specific manner: adenine (A) with thymine (T) and cytosine (C) with guanine (G). These base pairs form the rungs of the ladder, while the deoxyribose sugar and phosphate groups constitute the backbone.
This double-stranded structure confers extraordinary stability and flexibility to DNA. The hydrogen bonds between base pairs keep the strands tightly bound, ensuring the integrity of genetic information. Moreover, the double helix allows for DNA replication, the process by which new copies of DNA are synthesized. During replication, the strands “unzip”, and each serves as a template for the synthesis of a complementary strand, ensuring faithful transmission of genetic material.
The double helix also plays a crucial role in DNA repair. When DNA is damaged by environmental factors or replication errors, specialized enzymes recognize and correct the defects, relying on the complementary strand as a reference. This intricate repair mechanism ensures that genetic information is preserved accurately.
Furthermore, the double helix shape provides a protective shield for DNA. The outer layer of phosphate groups creates a negatively charged surface that repels destructive molecules and prevents DNA degradation. The compact structure of the double helix also minimizes exposure to potentially damaging agents.
In conclusion, the double helix structure of DNA is not merely an aesthetic feature; it is a masterpiece of molecular engineering that enables DNA’s vital functions: storage, transmission, and protection of genetic information. This iconic shape underlies the very essence of life, ensuring the continuity and integrity of our genetic heritage.