Decoding The Dna Blueprint: Understanding The Language Of Life
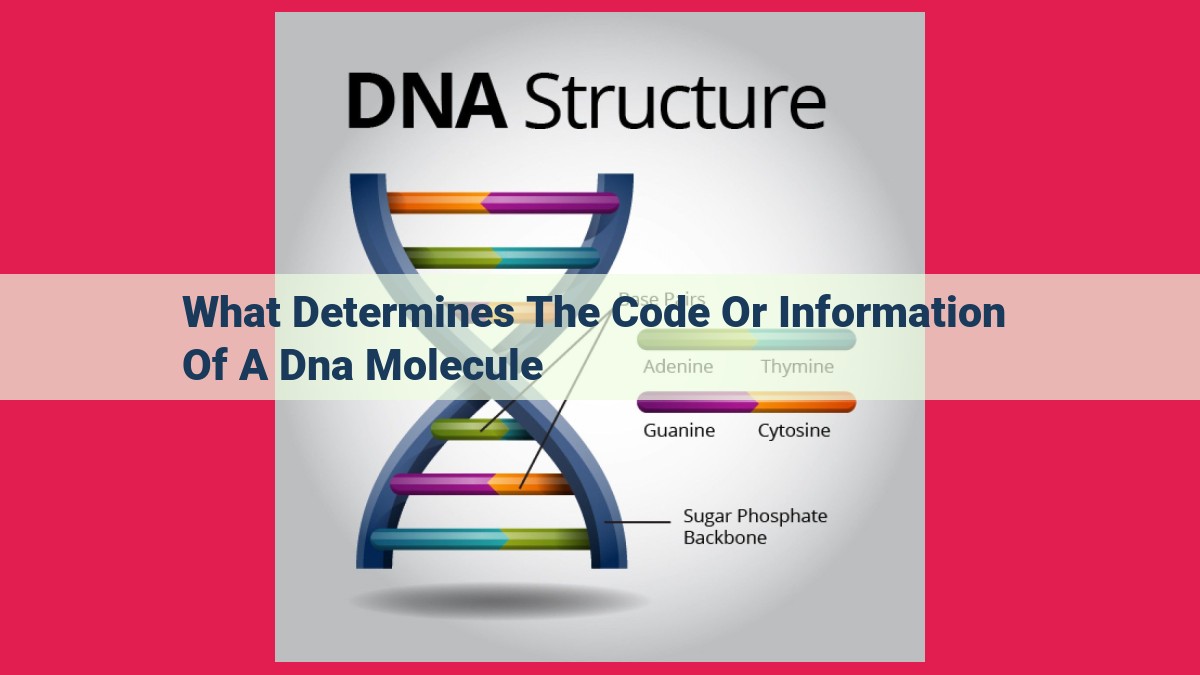
The code of a DNA molecule is determined by its sequence of nucleotides, which are composed of a nitrogenous base (adenine, cytosine, guanine, or thymine), a sugar (deoxyribose), and a phosphate group. The sequence of these nucleotides forms genes, which encode proteins or RNA molecules. The organization of genes into chromosomes provides stability and ensures orderly inheritance. The entire set of DNA in an organism is known as its genome, which contains the instructions for all biological processes.
The Building Blocks of DNA: Unveiling the Secrets of Nucleotides
In the realm of molecular biology, where lies the blueprint of life, we find the captivating molecule known as DNA. DNA, deoxyribonucleic acid, is the fundamental building material of heredity, encoding genetic information that governs our existence. At the heart of this remarkable molecule lies a fundamental unit, the nucleotide.
Composition of Nucleotides
Each nucleotide is a trio of components: a nitrogenous base, a deoxyribose sugar, and a phosphate group. The nitrogenous bases, adenine (A), cytosine (C), guanine (G), and thymine (T), are the true stars of the genetic code. These nitrogenous bases determine the genetic information carried by DNA.
Role in the Genetic Code
Nitrogenous bases pair with each other according to specific rules: A with T, and C with G. These base pairs form the rungs of the iconic double helix structure of DNA. The sequence of these base pairs, much like letters in a word, spells out the genetic code, providing instructions for the development and functioning of our bodies.
Unveiling the Genetic Code
The sequence of nucleotides in DNA determines the genetic traits of an organism. This sequence is meticulously read and translated by cells to produce proteins, the workhorses of our bodies, and other essential molecules. Nucleotides, therefore, serve as the fundamental units that orchestrate the intricate symphony of life.
The Sequence of Life: Base Sequence
In the realm of genetics, the sequence of nucleotides holds the key to unlocking the secrets of life. It’s not just the presence of the building blocks of DNA (nucleotides) but their precise arrangement that determines the genetic code unique to each organism.
Think of the nucleotides as individual letters. When sequenced correctly, they form words, which in turn form meaningful sentences. In DNA, these “sentences” encode the instructions for building proteins, the workhorses of our cells. This genetic information allows organisms to inherit traits, develop their characteristics, and maintain their species.
The sequence of nucleotides also contributes to the structure of DNA. Through base pairing, complementary nucleotides like adenine (A) and thymine (T), or cytosine (C) and guanine (G), bind together to form the famous DNA double helix. This structure protects the delicate genetic code from damage.
Unlocking the mysteries of the base sequence is the foundation for understanding genetics. It enables us to unravel the genetic basis of diseases, predict inherited traits, and even engineer new therapies. By deciphering the language of life, we gain a deeper appreciation for the intricate dance that makes life possible.
Units of Genetic Information: Meet the Genes
Unlocking the secrets of life requires us to delve into the intricate world of genetics. Central to this journey are genes, the fundamental units of heredity that carry the blueprint for our existence. These tiny segments of DNA hold the power to shape our traits, dictate our destiny, and even influence our health.
Defining Genes
Genes are specific regions within a DNA molecule that encode the genetic instructions for assembling proteins or RNA molecules. These proteins and RNAs play crucial roles in determining our physical and biochemical characteristics, from eye color to immunity.
Alleles: The Variations Within
Genes often exist in different forms called alleles. Alleles are variations of a gene that occur at the same location (locus) on homologous chromosomes. These variations can lead to different versions of the same protein or RNA molecule, influencing the phenotypic expression of a trait.
Genetic Variation and Alleles
Alleles are the driving force behind genetic variation. The diverse combinations of alleles within a population allow for a wide range of phenotypic variations, from subtle differences in height to complex diseases. This variation is essential for adaptation, survival, and the evolution of new species.
Genes are the building blocks of heredity, the gatekeepers of our genetic heritage. They govern our traits, shape our destiny, and connect us to the broader tapestry of life. Understanding genes is fundamental to unraveling the complexities of biology and paving the way for future advancements in medicine and biotechnology.
The Organized Archives: Chromosomes
In the world of genetics, chromosomes emerge as the custodians of our genetic legacy. Picture them as meticulously organized libraries, each tome containing a wealth of information essential for life’s symphony.
These biological marvels vary in size and shape, reflecting the complex genetic blueprints they harbor. Inside these archives reside genetic components known as genes, the enigmatic sequences responsible for dictating our traits, from eye color to disease susceptibility.
The centromere acts as the chromosome’s “control center,” ensuring the equal distribution of genetic material during cell division. This tiny region holds the threads that align and separate sister chromatids, identical strands that arise from DNA replication.
At the tips of chromosomes lie the telomeres, protective caps that prevent genetic erosion with each cell division. These “guardians of time” maintain the stability and integrity of our genetic code.
Chromosomes, like well-organized libraries, house the secrets of life, safeguarding our genetic past and shaping our future. Their intricate arrangement allows for the precise transmission of genetic information, ensuring the continuity of countless generations.
The Genome: The Complete Genetic Picture
In the realm of genetics, the genome reigns supreme as the blueprint of life. It holds the key to our unique traits, from the color of our eyes to our susceptibility to diseases. This colossal repository of genetic information resides within the nucleus of every cell, packaged into structures called chromosomes.
Chromosomes are thread-like bundles of DNA, each containing a specific set of genes. These genes are segments of DNA that encode instructions for making proteins, the workhorses of our bodies. Genes come in pairs, with each one occupying a specific locus on a chromosome. These pairs of genes are called alleles, and they may vary slightly from each other, contributing to the genetic diversity within a species.
The genome varies in size and complexity across organisms. Haploid genomes, found in reproductive cells like sperm and eggs, contain a single copy of each chromosome. Diploid genomes, found in non-reproductive cells, possess two copies of each chromosome, one inherited from each parent. Polyploid genomes, present in some plants and animals, contain multiple sets of chromosomes.
The genome is a dynamic entity, constantly undergoing changes through processes like mutation and recombination. These changes can introduce new genetic variations that drive evolution. The study of genomes, known as genomics, is revolutionizing our understanding of life, opening doors to new advancements in medicine, agriculture, and biotechnology.
Translating the Genetic Code: From Nucleotides to Amino Acids
Imagine the human genome as a vast library, filled with volumes of genetic instructions. These instructions, written in the language of nucleotides, guide the construction and function of every cell in our bodies. But how do these tiny building blocks translate into the complex proteins that power our lives? Enter the genetic code.
The genetic code is a set of rules that dictate how sequences of nucleotides (the building blocks of DNA) specify the order of amino acids (the building blocks of proteins). Each amino acid is encoded by a unique codon, which consists of three nucleotides. For example, the codon TAC codes for the amino acid tyrosine.
Start and stop codons are special sequences that mark the beginning and end of protein-coding regions. The start codon AUG signals the start of protein synthesis, while UAA, UAG, and UGA serve as stop codons, indicating that protein synthesis is complete.
The genetic code is universal among all living organisms, meaning that the same codon will always code for the same amino acid, regardless of the species. This universality allows scientists to compare and contrast genes from different organisms, opening up new avenues of research into evolution and disease.
Understanding the genetic code is crucial for uncovering the secrets of life and developing new therapies for genetic disorders. By deciphering the language of nucleotides, we gain a deeper understanding of the intricate workings of our cells and the potential to improve human health
From DNA to RNA: The Transcription Process
In the intricate realm of molecular biology, DNA stands as the blueprint of life, holding the genetic code that governs every aspect of an organism. However, this code cannot be directly translated into the proteins that perform vital functions within our cells. Instead, it must first be transcribed into a messenger molecule: RNA.
The Role of RNA Polymerase
Transcription is a complex process catalyzed by an enzyme known as RNA polymerase. This molecular machine binds to specific regions of DNA called promoter regions, which signal the start of a gene. Once bound, RNA polymerase unwinds the DNA double helix, exposing the nitrogenous bases that make up the genetic code.
Building the RNA Messenger
Using the DNA template, RNA polymerase begins synthesizing a complementary strand of RNA called messenger RNA (mRNA). This strand is composed of nucleotide bases that pair with their counterparts on the DNA strand. Adenine (A) pairs with uracil (U), cytosine (C) pairs with guanine (G), and so on. As the mRNA strand grows, it carries a precise copy of the genetic information encoded in the DNA.
The Journey of mRNA
Once the mRNA transcript is complete, it detaches from the DNA template and embarks on a journey to the ribosome, the protein factory of the cell. This journey may take place within the nucleus, where transcription occurs, or in the cytoplasm, where the ribosomes reside. Along the way, the mRNA transcript undergoes modifications that prepare it for translation, the process by which proteins are synthesized.
Decoding the Genetic Blueprint: Translation
Imagine DNA as the instruction manual for life, a complex code that contains the blueprint for every living organism. But how does this code translate into the proteins that build our bodies and govern our functions? This is where the process of translation comes into play.
Translation is the final step in the central dogma of molecular biology, the process that converts genetic information from DNA to RNA to protein. It’s a complex yet elegant dance involving intricate molecular machinery and a precise genetic code.
The Translation Machinery
The stage for translation is the ribosome, a large molecular complex that sits in the cytoplasm of the cell. Ribosomes are made up of two subunits, a large and a small subunit, which come together to form a tunnel-like structure. This tunnel is where the mRNA molecule, transcribed from DNA, enters and becomes the template for protein synthesis.
Transfer RNA: The Code Decipherers
Transfer RNA (tRNA) molecules are the key players in deciphering the genetic code. Each tRNA molecule has two important components: an anticodon and an amino acid attachment site. The anticodon is a short sequence of three nucleotides that is complementary to a specific codon on the mRNA molecule. The amino acid attachment site, on the other hand, carries amino acids, the building blocks of proteins.
Decoding the mRNA Codons
As the mRNA molecule passes through the ribosome tunnel, its codons are exposed one by one. The anticodon of a tRNA molecule binds specifically to the complementary codon on the mRNA. When a codon is matched, the corresponding amino acid is transferred from the tRNA’s attachment site to the growing polypeptide chain.
Codon by Codon, Protein by Protein
This process continues codon by codon, tRNA molecule by tRNA molecule, until a stop codon is reached. Stop codons do not code for any amino acids but signal the end of protein synthesis. The completed polypeptide chain is then released from the ribosome and folds into its functional protein structure.
The Precision of Translation
Translation is a remarkably precise process, ensuring the accurate synthesis of proteins. The genetic code is read in a specific order, and errors in codon recognition or tRNA binding can lead to the production of non-functional proteins.
So, the process of translation is the final step in the conversion of genetic information into the proteins that are essential for life. It’s a testament to the intricate and elegant mechanisms that govern the very fabric of our being.