Diploid Cell In Humans: Genetic Diversity, Development, And Aneuploidy
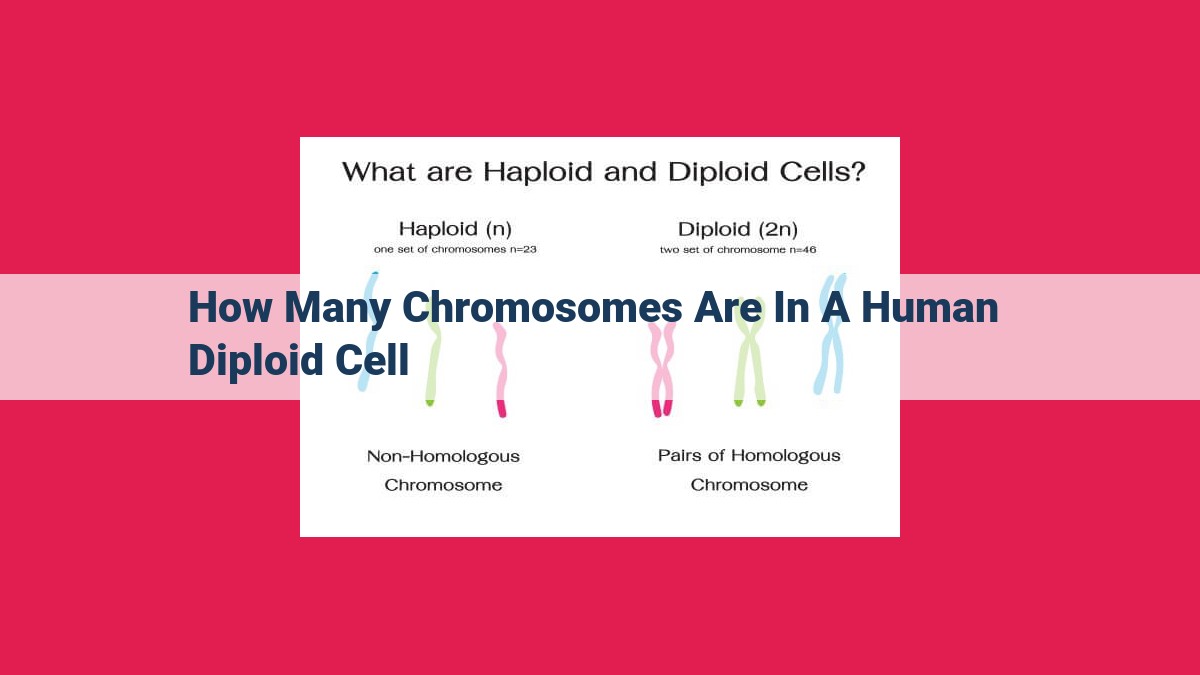
In humans, a diploid cell typically contains 46 chromosomes, arranged in 23 pairs. These chromosomes are inherited from both parents, with one set from each parent. This diploid number is essential for maintaining genetic diversity and ensuring the proper development and functioning of the organism. Deviations from this normal number, known as aneuploidy, can lead to various genetic disorders and developmental abnormalities.
What is a Diploid Cell?
In the tapestry of life, diploid cells paint a vibrant hue, carrying the genetic blueprints that define our very existence. To understand the cell cycle and the inheritance of traits from generation to generation, we must delve into the world of diploid cells.
Diploid cells are the bricks of our bodies, the building blocks from which the vast complexity of human life emerges. Diploid in Greek means “double,” and aptly so, as each of these cells contains two complete sets of chromosomes, one inherited from the mother and one from the father. These chromosomes are like miniature libraries, housing the genetic instructions encoded in DNA.
Diploid cells play a crucial role in maintaining the stability of our genetic make-up as they divide and reproduce. During cell division, the genetic material is meticulously duplicated and distributed equally to the daughter cells, ensuring that each new cell carries an identical complement of chromosomes. This process ensures the faithful transmission of genetic information from one generation of cells to the next.
The existence of diploid cells is of paramount importance for genetic inheritance. When diploid cells combine, such as during fertilization, they fuse their genetic material, creating a unique genetic tapestry that gives rise to future generations. This fusion of genetic material introduces new combinations of alleles, the alternative forms of genes, resulting in the vast diversity of traits that we observe in the living world.
**Understanding Chromosomes: The Key Players in Genetic Inheritance**
In the intricate world of cells, chromosomes stand as the custodians of our genetic legacy. These enigmatic structures, present in every cell of our bodies, are the blueprints for our unique traits and physical characteristics.
Chromosomes are organized, thread-like structures made primarily of deoxyribonucleic acid (DNA) and *proteins*. They are present in pairs within each cell. The size and shape of chromosomes vary depending on the specific species and cell type.
Within chromosomes, DNA is coiled around *proteins known as histones*. This highly organized arrangement protects the genetic information from damage. Each chromosome contains many genes, which serve as the fundamental units of inheritance. Genes carry instructions that dictate our physical and biochemical traits, from eye color to blood type.
The *structure of chromosomes* is dynamic, changing throughout the cell cycle. During cell division, chromosomes condense into visible structures, ensuring the equal distribution of genetic material to daughter cells. This intricate process is crucial for maintaining the correct chromosome number and ensuring the continuity of life.
Karyotype: Unveiling the Chromosome Blueprint
To truly comprehend the intricate dance of our genetic inheritance, we embark on a journey into the realm of karyotyping, a technique that unlocks the secrets of our chromosomes.
Karyotyping, like an artist’s keen eye, captures a snapshot of one’s chromosomal landscape. By artfully arranging and staining these tiny cellular blueprints, scientists paint a detailed portrait of an individual’s genetic makeup. This visual masterpiece holds invaluable information, revealing not only the number of chromosomes but also their structure and any potential abnormalities.
The significance of karyotyping is profound, extending far beyond the realm of scientific curiosity. In the realm of genetic counseling, karyotyping serves as a diagnostic tool, offering insights into the potential genetic risks that future generations may inherit. It empowers individuals with knowledge, empowering them to make informed decisions about their health and family planning.
Karyotyping also plays a pivotal role in prenatal diagnosis, safeguarding the well-being of unborn infants. By examining the chromosomes of a developing fetus, physicians can screen for potential genetic disorders, enabling timely interventions or providing crucial information for expectant parents.
Through the lens of karyotyping, we gain a deeper appreciation for the intricate symphony of our genetic inheritance. It’s a testament to the extraordinary complexity of life’s blueprint, meticulously inscribed within the threads of our chromosomes.
The Human Genome: A Blueprint of Life
Imagine a detailed instruction manual that contains the blueprint for creating and sustaining a human life. This manual, known as the human genome, is an intricate network of DNA that holds the secrets to our genetic makeup.
The human genome consists of approximately 3 billion base pairs of DNA, meticulously arranged in 23 pairs of chromosomes. Each chromosome is essentially a long strand of DNA, precisely coiled and packaged within the nucleus of every cell in our body. These chromosomes act as carriers of genetic information, encoding the instructions for the development and functioning of every aspect of our being, from our physical traits to our susceptibility to certain diseases.
The significance of the human genome cannot be overstated. It’s the key to understanding the fundamental biology that governs our lives. Through advancements in DNA sequencing technology and the field of genomics, scientists have gained unprecedented access to the intricacies of the human genome.
By decoding the genome, researchers have been able to map out the locations of genes and study their functions. This knowledge has revolutionized the fields of medicine, forensics, and biotechnology. It has enabled the development of personalized medicine, tailored to an individual’s genetic makeup, and has provided insights into the origins of diseases and the potential for gene therapies.
The human genome is a dynamic entity, constantly being studied and refined, as scientists delve deeper into its complexities. This ongoing exploration continues to unravel the mysteries of human life, offering new hope for improving our health and well-being.
Human Diploid Number: A Constant 46
In the realm of genetics, the human diploid number stands as a cornerstone of our genetic makeup. It refers to the precise number of chromosomes found in each cell. For humans, this magical number is 46, meticulously divided into 23 pairs.
Maintaining this constant diploid number is crucial for our well-being. Each chromosome carries a treasure trove of genetic information inherited from our parents, serving as the blueprint for our traits and characteristics. Altering this delicate balance can lead to developmental abnormalities and genetic disorders.
The human diploid number is steadfastly preserved throughout our development, from the moment of conception to the twilight of our years. During cell division, the chromosomes meticulously replicate and segregate to ensure that each new cell receives its precise complement of 46 chromosomes. This intricate process safeguards our genetic heritage, ensuring that the information encoded within our DNA remains intact.
Homozygous and Heterozygous: Unraveling the Secrets of Allelic Variations
In the realm of genetics, the concept of alleles unveils a fascinating world of genetic diversity. Alleles are alternative forms of a gene that occupy specific locations on chromosomes, inherited from both parents. These variations give rise to the unique traits that distinguish individuals within a population.
Homozygosity, a condition where an individual possesses two identical alleles for a specific trait, ensures consistency in the expressed phenotype. This is because both alleles provide identical instructions for protein synthesis, resulting in a consistent observable characteristic.
Conversely, heterozygosity arises when an individual harbors two different alleles for a particular trait. This genetic diversity introduces variability into the phenotypic expression. One allele may be dominant, masking the effect of the recessive allele, while in other cases, both alleles exert their influence, leading to intermediate or blended traits.
The interplay between homozygous and heterozygous individuals plays a crucial role in inheritance patterns. For example, a homozygous dominant individual will always pass on the dominant allele to their offspring, while a homozygous recessive individual will only transmit the recessive allele. Heterozygous individuals, however, possess the potential to pass on either allele, leading to more diverse genetic outcomes within families.
Understanding allelic variations is essential for comprehending the genetic basis of traits, diseases, and evolutionary processes. By studying the inheritance patterns of homozygous and heterozygous individuals, scientists can gain valuable insights into the molecular mechanisms underlying human health and diversity.
Autosomes vs. Sex Chromosomes: A Matter of Gender
In the realm of genetics, chromosomes play a pivotal role in determining our traits and characteristics. Among these chromosomes, autosomes and sex chromosomes stand out due to their unique functions.
Autosomes: The Workhorses of Inheritance
Autosomes are the non-sex chromosomes that come in pairs. Every human cell contains 22 pairs of autosomes, each pair carrying genes that influence various aspects of our physical traits, such as eye color, hair texture, and height.
Sex Chromosomes: Guardians of Genetic Sex
In contrast to autosomes, we inherit only one pair of sex chromosomes, denoted as X and Y. These chromosomes hold genes responsible for determining our genetic sex. Females typically possess two X chromosomes (XX), while males carry one X and one Y chromosome (XY).
The Y chromosome is smaller than the X chromosome and carries few genes. Its primary role is to trigger the development of male reproductive structures and the production of sperm. The X chromosome, on the other hand, is larger and carries genes involved in a wider range of traits, including blood clotting and color vision.
Sex-Linked Traits: Carried on the X Chromosome
Genes located on the X chromosome are referred to as sex-linked genes. Since males have only one X chromosome, they express any traits associated with these genes directly. Females, with their two X chromosomes, may be homozygous (carrying two copies of the same allele) or heterozygous (carrying different alleles) for sex-linked traits. This can lead to interesting inheritance patterns and the potential for certain traits to be more common in one sex than the other.
For example, red-green color blindness is a sex-linked trait caused by a recessive allele on the X chromosome. If a male inherits this allele from his mother, he will be color blind. A female, however, needs to inherit two copies of the recessive allele (one from each parent) to exhibit color blindness. This is why color blindness is more prevalent in males than in females.
Aneuploidy: When Chromosome Numbers Go Wrong
In the realm of genetics, chromosomes play a pivotal role in carrying the blueprint of life. Each cell within our bodies houses a specific number of chromosomes, ensuring the proper development and functioning of our physical and biological systems. However, sometimes, this delicate balance can be disrupted, leading to a condition known as aneuploidy.
Aneuploidy occurs when a cell has an abnormal number of chromosomes. This can happen due to errors during cell division, where chromosomes are not distributed equally between daughter cells. The consequences of aneuploidy can be wide-ranging, depending on the type and severity of the chromosomal imbalance.
Types of Aneuploidy
Aneuploidy can manifest in various forms, each with its own potential consequences:
-
Trisomy: This occurs when a cell has an extra copy of a particular chromosome. Common examples include Down syndrome (trisomy 21) and Patau syndrome (trisomy 13). These conditions can cause developmental delays, physical abnormalities, and health complications.
-
Monosomy: In monosomy, a cell is missing a copy of a specific chromosome. Turner syndrome (monosomy X) is a known example, which can lead to growth difficulties, delayed development, and infertility in females.
Consequences of Aneuploidy
The effects of aneuploidy depend on the specific chromosome involved and the number of copies present. Some aneuploidies can be lethal, while others may result in varying degrees of physical and cognitive impairments. In certain cases, aneuploidies can also contribute to an increased risk of certain cancers and reproductive difficulties.
Impact on Pregnancy
Aneuploidy is a major concern in prenatal diagnosis. Screening tests can detect chromosomal abnormalities in developing fetuses. Early detection allows for informed decisions regarding pregnancy management and helps prepare for potential health challenges.
Understanding Aneuploidy
Aneuploidy is a complex genetic condition that can have profound implications for individuals and families. By understanding the mechanisms behind aneuploidy and the potential consequences, we can better address the challenges associated with it. Continued research and advancements in genetic technology hold promise for improving our ability to diagnose, manage, and potentially prevent aneuploidy in the future.